Startling Snapshots of Science
Vibrant Images Push Forward Environmental Health Research
Scientists and artists obviously don’t spend their days doing the same thing, but that doesn’t mean the former can’t sometimes inadvertently be the latter. Take for example the 14 IRP research fellows who submitted images to the most recent edition of the Fellows Scientific Image Competition at NIH’s National Institute of Environmental Health Sciences (NIEHS). The competition, held every few years, shows that biomedical science can not only save lives, but also look good doing it.
“We are excited to showcase the work of our trainees using visual media, which reminds us there is art in science and showcases the important research we’re all engaged in at NIEHS,” says NIEHS Scientific Director Darryl Zeldin, M.D., who initiated the competition.
Read on to see a small sample of the images featured in this year’s competition and learn about how they are helping IRP researchers figure out the ways environmental exposures affect the brain, reproductive system, lungs, and other parts of our bodies.
A Plethora of Pregnancy-Supporting Cells
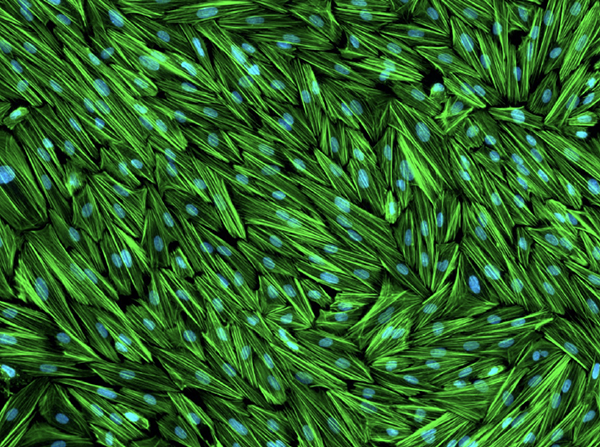
The above image may at first glance look like some kind of mat made from weaving together strands of grass — a mat that someone then spilled blue paint on. In reality, it shows a tight-knit network of ‘stromal’ cells from the human endometrium, the tissue that lines the inside of the uterus. The green strands represent a protein called filamentous actin, or F-actin for short, which helps the endometrium maintain its structure. The blue dots, on the other hand, mark the endometrial cells’ nuclei.
IRP postdoctoral fellow Ryan Marquardt, Ph.D., exposed these cells to a specific mix of hormones for three days in order to “mimic the hormonal environment encountered by endometrial stromal cells during the window of implantation, the critical time period when an embryo is able to implant in the uterus and receive the support needed to maintain pregnancy,” he explains. Among their many effects on the endometrium, those hormones re-shape the F-actin fibers that bind endometrial stromal cells together. By studying those cells and the F-actin fibers that link them, he and his fellow scientists in the lab of IRP senior investigator Francesco DeMayo, Ph.D., hope to learn how exposure to certain substances might contribute to infertility and pregnancy complications, since interference with endometrial stromal cells can reduce the chances of an embryo successfully implanting in the uterus or surviving until it is born.
“Chronic reproductive diseases such as endometriosis and endometritis cause long-term pain and fertility problems, placing a heavy burden on patients who suffer from them and on the medical system,” Ryan says. “Cell models like this one enable us to perform controlled experiments in the lab aimed at understanding how the uterus works in healthy conditions and how environmental insults disrupt its function and cause disease.”
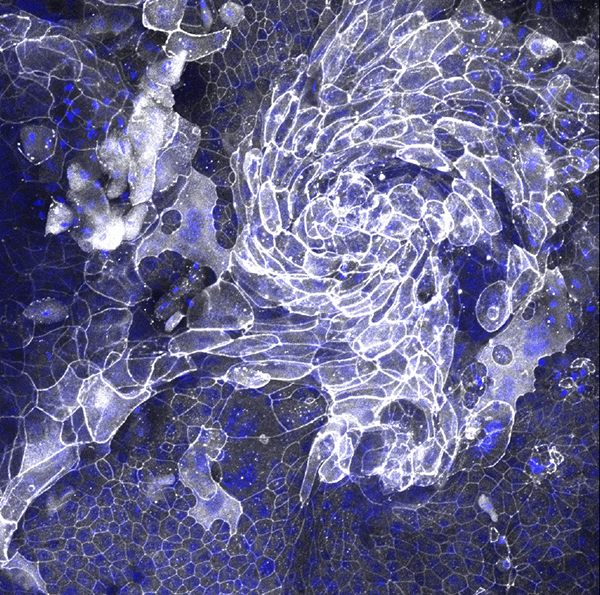
The resemblance of this image to a hurricane is appropriate, since it shows a set of epithelial cells from a mouse’s trachea — basically, the cells that line its airway. The image was submitted to the competition by Jonas Ritter, a medical student in the lab of IRP senior investigator Stavros Garantziotis, M.D. The blue dots show the cells’ nuclei, while white represents a protein called ZO1 that is part of what scientists call ‘cell junctions.’
“Cells that line the body’s surfaces — like the skin, airway, or intestines — must form tight connections with each other so they become impenetrable to environmental particles like microbes or pollutants,” explains Dr. Garantziotis. “To do this, they use cell junction proteins that ‘button’ with each other and bind the cells together.”
These particular cells come from a mouse lacking the TLR5 gene, which contains the instructions for making a receptor on the surface of cells that recognizes bacterial proteins. Dr. Garantziotis’ lab has found that the TLR5 receptor is important for protecting the cells in our airway from the bacteria we routinely breathe in. However, between 3 and 10 percent of people lack functional TLR5 receptors, just like the mice his lab is studying, and as a consequence they are more likely to develop lung diseases like lung cancer or a scarring-like phenomenon called fibrosis. It’s not surprising, then, that the TLR5-deficient cells in the image don’t look the way healthy cells are supposed to.
“When cells are unhealthy or injured, they can change into a different shape in order to try to protect themselves from further injury,” Dr. Garantziotis says. “This is called metaplasia. In this case, the airway cells that are normally smooth and tender — think of the surface inside your nose, for example — have become skin-like, thickened and rough. This is called squamous metaplasia. In the lung, squamous metaplasia happens in smokers, for example, and can precede the development of lung cancer. Therefore, it is important to understand what causes squamous metaplasia and how to prevent it,” in order to come up with ways to stop bacterial infections, smoking, or pollution from harming the lungs of people without TLR5.
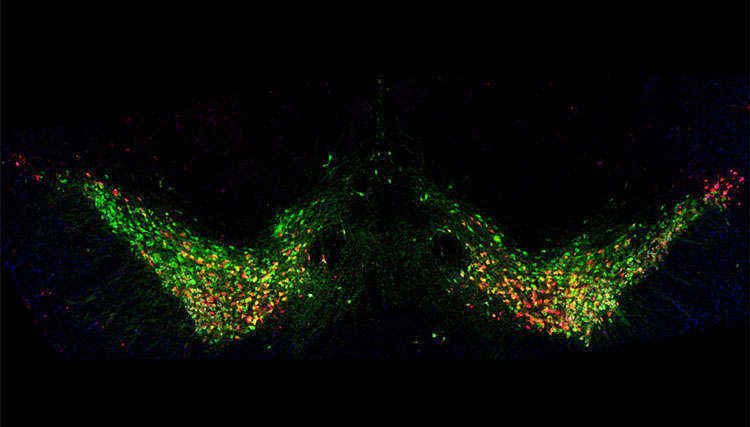
One of the reasons that even very young children know to expect presents when they see red and green lights strewn around the house is because of neurons in the brain that send signals using a chemical called dopamine. The brain uses dopamine to help link rewarding experiences like opening presents with certain occurrences. And since we typically have to take some sort of action to gain a reward when a related situation occurs, it’s not surprising that dopamine is also a key part of how the brain controls movements.
Unfortunately, that means that when neurons that produce dopamine die, it reduces our ability to control the way our body moves. And that is precisely what happens in Parkinson’s disease.
Sukanya Saha, Ph.D., a postdoctoral fellow in the lab of IRP senior investigator Guohong Cui, M.D., Ph.D., produces images like the one above during experiments that reveal what happens to those ‘dopaminergic’ neurons in patients with Parkinson’s disease. In that specific image, neurons from a mouse brain that contain an enzyme called tyrosine hydroxylase (TH) have been made to glow green because neurons use that enzyme to make dopamine. The red neurons, on the other hand, are neurons that have been infected by a benign virus administered to the mice that is designed to deliver a gene for that red marker to the animals’ dopaminergic neurons. Consequently, the overlap of red and green denotes dopaminergic neurons that have been infected by that virus, confirming that the virus can successfully deliver a gene to those neurons and that the neurons subsequently start making the protein that the gene codes for.
Having confirmed that, Dr. Saha proceeded to using a virus that delivers a more interesting gene into dopaminergic neurons — a gene that contains a mutation linked to early-onset Parkinson’s disease in humans. The mice involved in that experiment were also exposed to some sort of environmental condition thought to contribute to the development of Parkinson’s.
“We tried to create a ‘double-hit’ Parkinson’s model with both genetic and environmental insults,” Dr. Saha explains. “When we examined this region in the mice, most of those TH-positive neurons in green were lost. Thus, we successfully created a Parkinson’s mouse model,” which she and her colleagues can now use to study the disease’s development and figure out possible ways to treat it.
“To date, there are not many good animal models available to study Parkinson’s,” she continues, “so it is important to have a suitable Parkinson’s mouse model to learn about Parkinson’s and improve human health.”
A Red, White, and Blue Map of Scarring in the Lung

By now, you’ve probably caught on that scientific images are often not what they first appear to be — so no, this isn’t just an oddly shaped sliced of moldy Swiss cheese. It’s actually a slice of tissue from a mouse’s lung. This lung, however, is not in very good shape due to a scarring process known as fibrosis.
“You can see that the lung looks like a slice of Swiss cheese, and where you normally find the holes in the Swiss cheese in this image, you have the lung ‘air sacs,’” explains Wilfred Lopez Perez, Ph.D., a postdoctoral fellow in the lab of IRP senior investigator Michael Fessler, M.D. “These air sacs have a very thin layer that permits oxygen to move into the bloodstream. The sections of the image that are more purple and look compressed would be our fibrotic, or scarred, lung tissue.”
By using a specific staining technique to mark a structural protein called collagen that becomes more concentrated in areas where fibrosis has occurred, Dr. Lopez Perez is able to create a map of fibrosis in lung tissue. Such maps can then be compared to one another when taken from different mice involved in different experiments or from the same mouse at different points in time. In this way, Dr. Lopez Perez and his colleagues can assess how their experiments affect fibrosis in the animals’ lungs, providing important information that can be used to find ways to alleviate the condition in the many people who struggle to breathe due to the condition.
“In the case of the lung, which needs a very thin layer to receive oxygen, when fibrosis happens, you are left with thicker layers that do not permit the flow of oxygen into the blood,” Dr. Lopez Perez explains. “Patients that suffer from lung fibrosis develop difficulty breathing, dry cough, wheezing, and many other respiratory problems.”
“Many new patients develop lung fibrosis in the U.S. every year and there is no real cure for this disease,” he adds. “Better understanding of how lung fibrosis develops and progresses may lead to novel treatments that would help the thousands of patients battling with this disease.”
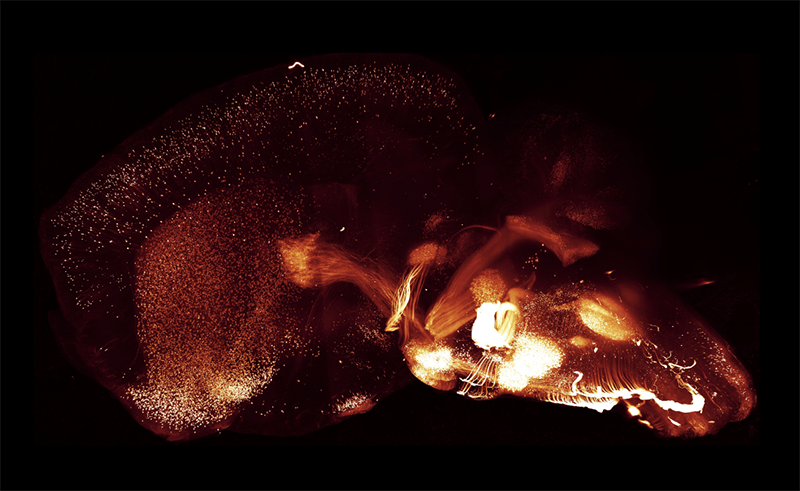
A lot of scientific terminology is baffling to most people, but every now and then, scientists pick a pretty straightforward name for something they invent or discover. That’s the case with a protein called tdTomato, which is just a fluorescent protein that glows in the same color as a ripe tomato.
In the above image, IRP postdoctoral fellow Oliver Goral, Ph.D., has used tdTomato to light up neurons in a mouse’s brain that use a chemical called acetylcholine to communicate with other cells. To do that, he and his colleagues in the lab of IRP senior investigator Jerry Yakel, Ph.D., created a genetically modified mouse that produces tdTomato only in those ‘cholinergic’ neurons. They can then use images like that one to count the number of cholinergic neurons in the animals’ brains and learn more about their characteristics when they are healthy. Such data will pave the way for future studies designed to answer questions about factors that might change the behavior or reduce the number of those cholinergic neurons, which are involved in a variety of important brain functions, including learning, movement, memory, and attention.
“This is a reference dataset which shows us how the system of cholinergic neurons looks like in the healthy brain,” Dr. Goral says. “In the future, we want to go ahead and test whether diseases or environmental exposures alter these cholinergic neurons in any way. Using this three-dimensional imaging approach, we would be able to compare the numbers or shape of the cholinergic neurons and know if they may be affected by exposures or disease.”

What might at first look like bursts of fireworks in a dark sky are actually two cross-sections of a mouse testis during the final stages of the mouse’s development in its mother’s womb. As pretty as these images are, though, using different colors to distinguish between the different types of cells in the testis has important practical applications as well.
“We used genetically engineered mice to make certain cells glow green at early stages of development, allowing us to track what those cells become later,” explains Martín Andrés Estermann, Ph.D., a postdoctoral fellow in the lab of IRP senior investigator Humphrey Yao, Ph.D. “To identify specific cell types, we also used special antibodies that detect proteins that are only specific to those cell types, which show up in magenta and red. By combining these techniques, we can track how specific cells develop over time. This approach, called cell lineage tracing, is a powerful tool in developmental biology to understand how organs and tissues form.”
In this case, the left image shows cells called Leydig cells in magenta, which secrete hormones called androgens. Androgens cause a mouse to develop typically male characteristics in the womb and, later in life, spur the production of sperm and the emergence of the male characteristics that appear as an animal becomes sexually mature, like a human teen’s deepening voice and facial hair.
The two images differ in what the color red represents. Red denotes a protein called smooth muscle actin in the image on the left, which is important for muscular contraction and plays a key role in pushing sperm from the testis to another part of a male mouse’s reproductive system, the epididymis, where the sperm mature. In the right image, on the other hand, the red marks cells containing a molecule called COUP-TFII, which acts as a genetic switch for the testis, controlling the activity of other genes. The combination of the red marker for COUP-TFII and a blue marker for cells’ nuclei creates the magenta seen in the image on the right.
Importantly, cells with COUP-TFII eventually transform into several other cell types as a mouse embryo develops, including the hormone-secreting Leydig cells. By manipulating the gene that contains the directions for making COUP-TFII, Nr2f2, Dr. Estermann and his colleagues in Dr. Yao’s lab hope to learn more about the different steps involved in sexual development and the consequences of variations in any of those steps.
“When we deleted Nr2f2 during development, we saw major disruptions in the formation of several types of cells,” he says. “Notably, the mice had fewer and less functional fetal Leydig cells. This led to testicular abnormalities such as underdeveloped, undescended testes and malformed genitals. These are typical features of differences of sex development, or DSDs for short, which are a range of conditions where human sex development differs from typical. Interestingly, these mouse characteristics closely mirror those observed in humans with mutations in Nr2f2.”
“Beyond identifying Nr2f2’s crucial role, our study also uncovered new genes and molecular pathways that may help improve the diagnosis and treatment of Nr2f2-related DSDs,” Dr. Estermann continues. “Overall, this work provides new insights into how the testis forms and functions and could have long-term impacts on understanding and managing reproductive health conditions in humans.”
Subscribe to our weekly newsletter to stay up-to-date on the latest breakthroughs in the NIH Intramural Research Program.
Related Blog Posts
This page was last updated on Monday, May 5, 2025