IRP Scientists Continue Efforts to Quell Pandemic
COVID-19 Research at NIH Show No Signs of Slowing
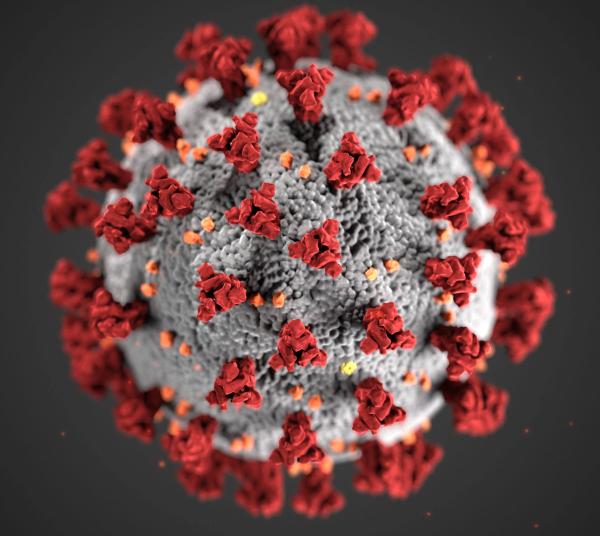
A special funding program, known as ITAC, has provided millions of dollars to IRP researchers studying the novel coronavirus that causes COVID-19.
It’s been more than two years since the first outbreak of COVID-19 occurred in China. During that time, amazing scientific advances have dramatically altered prevention and treatment for the illness, including the development of remarkably safe and effective vaccines. However, even with widespread vaccination, scientists predict that the disease will continue to circulate in society indefinitely, with seasonal ebbs and flows like the flu.
As a result, even as COVID-19 vaccine shots rolled out by the hundreds of millions, numerous IRP researchers continued studying the disease and the virus responsible for it. Many of these projects have been funded by the NIH’s Intramural Targeted Anti-COVID-19 Program (ITAC), an initiative that provides IRP researchers with funding for research related to COVID-19. Over the past year and a half, ITAC has provided more than $12 million to support a wide variety of projects — more than can be covered in just one blog post. Read on to learn about just a handful of the many ways IRP researchers are contributing to the fight against COVID-19, and stay tuned next week for another blog describing even more ITAC-funded COVID research.
Investigating the Novel Coronavirus’ Biology and Behavior
In many cases, developing treatments for an infectious disease begins by understanding what makes a bacteria, virus, or other pathogen tick. SARS-CoV-2, the virus that causes COVID-19, is certainly no exception.
While many researchers have focused on how the novel coronavirus gains entry into cells using its infamous spike protein, IRP senior investigator Nihal Altan-Bonnet, Ph.D., has been taking the opposite approach: exploring how the virus exits cells. All viruses use the cells they infect to make copies of themselves, but if those viral copies cannot leave the cell, they cannot go on to infect new ones.
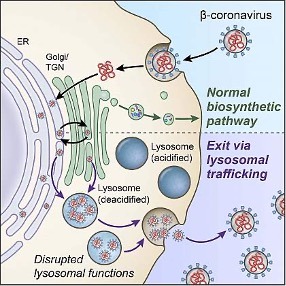
The components of the normal biosynthetic secretory pathway (top) and the lysosome pathway (bottom), which Dr. Nihal Altan-Bonnet’s lab discovered the SARS-CoV-2 virus uses to exit cells.
While most viruses escape from cells using the pathway that cells use to expel growth factors and hormones, known as the ‘biosynthetic secretory pathway,’ Dr. Altan-Bonnet’s lab discovered that SARS-CoV-2 exits cells via a cellular structure called the lysosome that cells use to break down and dispose of waste products. The virus accomplishes this by reducing the acidity of the environment inside the lysosome so that the lysosome cannot break it down, allowing the virus to escape the cell unscathed. What’s more, Dr. Altan-Bonnet’s team discovered that the way the virus affects lysosomes might also alter the way infected cells interact with the immune system, so her research could potentially help explain some of the immune system abnormalities seen in COVID-19 patients.
She plans to continue studying the novel coronavirus’ effects on lysosomes, including how the virus gets to the lysosomes from the part of the cell where it replicates and which molecules from the lysosome the virus takes with it when it exits the cell. Her lab is also searching for compounds that could help treat COVID-19 patients by blocking the virus’ ability to exit cells.
“Stopping the virus from getting into a cell, stopping it from replicating, and stopping it from getting out would be a multi-faceted, effective approach to get maximum antiviral benefits,” Dr. Altan-Bonnet says. “Hitting just one of these processes may not be as effective, as viruses mutate and escape from inhibition. By targeting all three, ideally all at once, you can stop the virus dead in its tracks and not give it an opportunity to escape by mutating.”
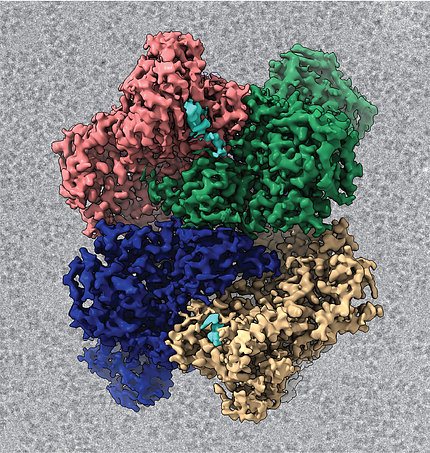
Image credit: Meredith Frazier
A structural image of the coronavirus Nsp15 protein that Dr. Robin Stanley’s lab is studying.
Meanwhile, the lab of IRP senior investigator Robin Stanley, Ph.D., hopes that new treatment approaches may come from learning more about how SARS-CoV-2 processes its own genetic material. Unlike humans, whose genome is encoded in double-stranded DNA, the SARS-CoV-2 genome is made up of single-stranded RNA. Part of that RNA contains the instructions for manufacturing ‘non-structural proteins,’ or Nsps, which help synthesize and process the virus’ RNA. One particular NSP, called Nsp15, processes viral RNA in a way that prevents our cells from recognizing it as non-human and breaking it down. Consequently, if scientists could stop Nsp15 from doing its job, cells might be able to stop the novel coronavirus from replicating inside them.
In 2021, Dr. Stanley’s team published the first detailed structural images of Nsp15 using a technique called cryo-electron microscopy. In addition, the researchers revealed the structure of Nsp15 when it is bound to RNA molecules in various stages of processing. They hope to use this structural knowledge of Nsp15 to design synthetic compounds that inhibit it, which could help COVID-19 patients by reducing the virus’ ability to make copies of itself inside their bodies.
Plunging Into Papillae to Explore COVID’s Effects on Taste
Early in the pandemic, as more and more people became infected with COVID-19, it became increasingly apparent that sometimes the only sign that someone had caught the disease was that food suddenly and inexplicably lost all flavor. While this distressing condition is temporary in many patients, others lose their senses of smell and taste for long periods of time, and some never regain them after recovering from the illness.
IRP senior investigator Josephine Egan, M.D., thought her lab’s focus on type 2 diabetes might help her solve the mystery behind these common symptoms. As part of its research, her lab studies a hormone called GLP-1, which is produced both in the digestive system and in the taste receptor cells on our tongue. These taste receptor cells are contained in taste buds, which themselves reside in specialized structures called taste papillae.
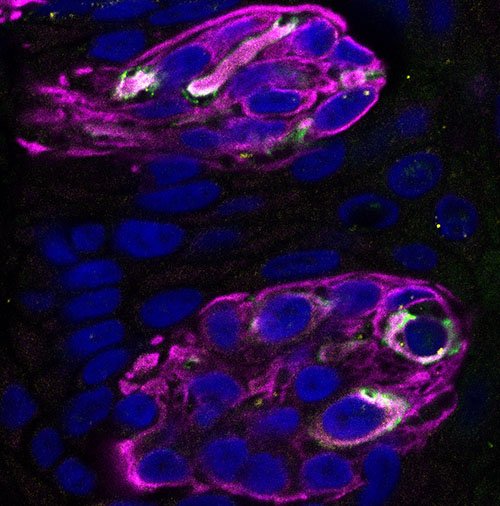
This image from Dr. Egan’s lab shows two taste buds (purple), each of which contains 50-60 taste receptor cells (blue cells surrounded by purple). The white areas mark the locations of ACE2 receptors.
“When COVID became a pandemic, we already had human biopsies of taste papillae containing taste buds in our lab,” Dr. Egan explains. “So we were in a unique position at the time.”
Dr. Egan’s team would go on to show that a subset of taste receptor cells have ACE2 on their surfaces, the molecule that SARS-CoV-2’s spike protein binds to so the virus can infect cells. Her lab also found the virus replicating inside of these taste receptor cells. Now, the group is conducting experiments to learn how exactly the virus affects the taste receptor cells it infects. Since our tongues repeatedly come into contact with food, taste receptor cells are constantly being damaged and replenished, and Dr. Egan’s group has found preliminary evidence that SARS-CoV-2 may impair this process. The team has also identified signs of inflammation within infected taste papillae that could contribute to COVID patients’ taste-related symptoms.
Assessing the Damage Wrought by COVID-19
When figuring out how to combat any illness, it’s crucial to know what it does to the human body. This is especially important for COVID-19, whose effects are so wide-ranging and variable that nearly a million Americans have died from the disease while tens of millions have had only mild symptoms or no symptoms at all.
IRP senior investigator Anthony Suffredini, M.D., is one of many NIH scientists who have jumped into this area of COVID research. For years, Dr. Suffredini’s group at the NIH Clinical Center has tracked how infections — particularly by bacteria and fungi — affect the lungs using a combination of imaging tools and a procedure called bronchoalveolar lavage (BAL), which doctors use to collect cells and molecules from abnormal areas in the lungs.
“It was an easy move to use these methods to study the clinical course of SARS-CoV-2 infection,” he says.
Since May of 2020, Dr. Suffredini’s team has enrolled more than 125 COVID-19 patients in his study, some of whom visited the NIH Clinical Center and some of whom were hospitalized at medical centers in Washington, D.C. The collaborations with the D.C. hospitals were particularly important for ensuring the study could successfully recruit individuals from groups at high risk for severe COVID-19, such as patients from minority groups and people who speak little or no English. The patients in Dr. Suffredini’s study undergo an array of procedures, including BAL, blood draws, tests of lung function, high-resolution computerized tomography (CT) scans of their lungs, magnetic resonance imaging (MRI) scans of their brains, and imaging of their hearts and lungs using an advanced ‘low-field’ MRI scanner at the NIH Clinical Center, one of only three such scanners currently available for clinical use. The low-field MRI scanner, made available to Dr. Suffredini’s team through a collaboration with NIH’s National Heart, Lung, and Blood Institute (NHLBI), can simultaneously produce structural scans of the lungs and images showing the oxygen levels and blood flow in different parts of the organ.
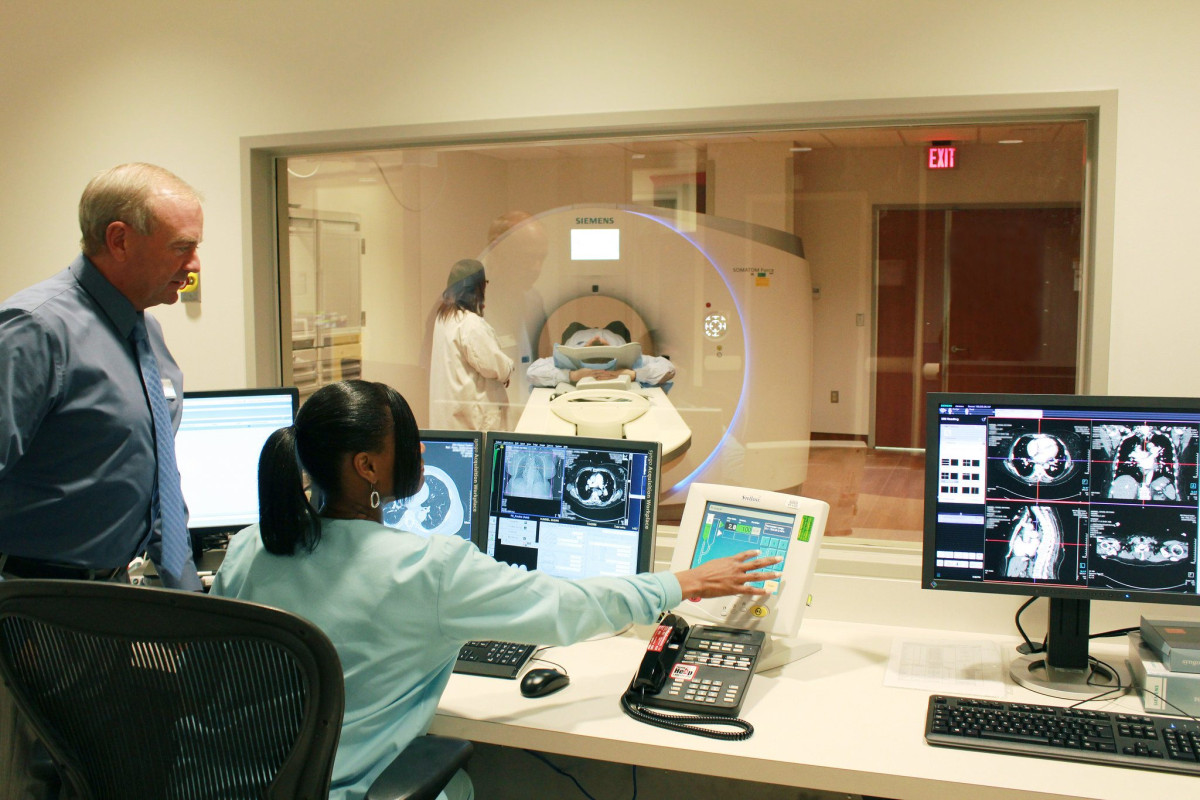
A patient undergoes a CT scan at the NIH Clinical Center.
Using these tools, the IRP researchers have observed that a small fraction of COVID-19 patients have scarring in their lungs, known as pulmonary fibrosis. On the other hand, the team has not found much in the way of heart problems in the study’s participants so far.
Dr. Suffredini and his colleagues are currently assessing changes in the levels of various proteins in patients’ blood and the samples collected via BAL, as well as examining whether the infection appears to have any effects on patients’ brains. They will also continue to follow patients in the second and third years after their infections in order to track the long-term effects of COVID-19. Ultimately, the project hopes to provide a comprehensive picture of the disease’s effects throughout the body, which could help point to better ways to understand the process of damage and recovery in different organs. This information also may help in the development of additional therapies for COVID-19.
While studying how COVID-19 affects otherwise healthy people is a crucial area of investigation, many people with pre-existing conditions have been catching the illness as well. People with autoimmune diseases, in which the immune system attacks the body, could provide useful insights into how the immune system responds to the virus and the vaccines that prevent it, for example. This, in turn, could help doctors improve treatment for COVID-19 patients with and without autoimmune conditions.
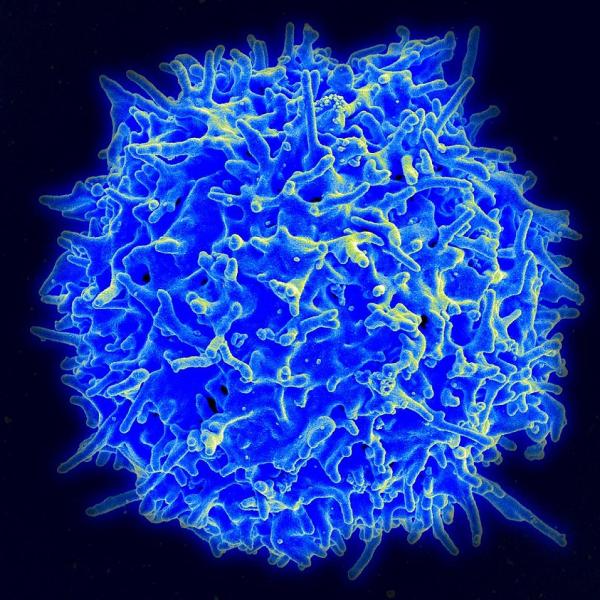
T cells like this one play an important role in both the healthy immune system and in autoimmune diseases like those Dr. Mariana Kaplan studies.
To that end, IRP senior investigator Mariana Kaplan, M.D., is applying her decades of experience studying autoimmune diseases to the study of COVID-19 in people with such ailments. Her lab’s ongoing study is comparing how COVID-19 infection and vaccination affect various types of immune cells, disease-fighting antibodies, and immune-system molecules called cytokines present in the blood of people with and without autoimmune diseases. Dr. Kaplan’s team is also examining how the SARS-CoV-2 virus and COVID-19 vaccines affect blood vessels in patients with autoimmune diseases.
If the researchers can pinpoint how the effects of the vaccine or the virus itself are different in people with those disorders, the findings could provide clues as to which specific switches in the immune system the virus trips and how it harms blood vessels, providing potential targets for therapies that influence the immune system’s response to the virus and reduce the damage it causes. Moreover, since abnormal immune system responses and damage to blood vessels may underlie some of the complications seen in people with long-COVID, Dr. Kaplan’s research may eventually benefit patients who are living with that mysterious syndrome.
As the emergences of the Delta and Omicron variants have shown, the novel coronavirus continues to hold new surprises for researchers and public health officials. Nevertheless, the ongoing efforts of IRP researchers offer hope that we will be ready to deal with whatever new twists and turns the pandemic takes in the future.
Subscribe to our weekly newsletter to stay up-to-date on the latest breakthroughs in the NIH Intramural Research Program, and don’t forget to check back next week for part 2 of our coverage of the IRP’s ongoing COVID research.
Related Blog Posts
This page was last updated on Tuesday, May 23, 2023