Colleagues: Recently Tenured
Meet your recently tenured colleagues: Alexander Chesler (NCCIH), Dima Hammoud (CC), Zayd M. Khaliq (NINDS), James Kochenderfer (NCI-CCR), Jadranka Loncarek (NCI-CCR), Wei Lu (NINDS), Nehal Mehta (NHLBI), Jordan Meier (NCI-CCR), Anant Parekh (NIEHS), and Armin Raznahan (NIMH)
ALEXANDER CHESLER, PH.D., NCCIH
Senior Investigator and Head, Sensory Cells and Circuits Section, National Center for Complementary and Integrative Health
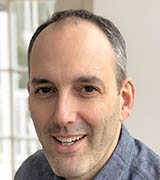
Education: Bard College, Annandale-On-Hudson, New York (B.A. in biology); Columbia University, New York (Ph.D. in biology)
Training: Postdoctoral training at the University of California at San Francisco (San Francisco)
Came to NIH: In 2013 as a Stadtman Investigator
Outside interests: Drinking coffee; cooking; traveling; hiking; camping
Website: https://irp.nih.gov/pi/alexander-chesler
Research interests: The central question guiding my research is how sensory input is detected and processed by the brain to evoke experiences and behaviors. My work focuses on identifying peripheral somatosensory neurons tuned to specific types of stimuli (such as touch, temperature, and pain), the molecules they use for transduction, and the neural circuits that they activate. Through my research I seek to understand the basis by which some stimuli are perceived as innocuous while others noxious, and how these distinctions are modulated by someone’s physiological state or prior experience. The hope is that improving our knowledge of these basic mechanisms will be useful in developing new therapeutic approaches for treating acute and chronic pain.
My group uses mouse genetics, electrophysiology, in vivo imaging, and behavior to study how stimuli are detected and encoded. Together, these approaches help us to better understand the importance of specific molecules for the responses of defined classes of sensory neurons and to map neural pathways for touch and pain. In parallel, together with Carsten Bönnemann’s group (National Institute of Neurological Disorders and Stroke), we have identified and characterized patients with a rare inherited disorder that selectively affects mechanosensation (the process by which mechanical stimuli are translated into neuronal impulses) due to damaging mutations in the gene for the stretch-gated ion channel PIEZO2. Studying these patients allows us to probe basic questions about the senses of touch, proprioception (sense of body position), interoception (sense of the internal state of the body), and mechanical pain. Through our research, we are gaining insight into human experiences that, by definition, are impossible to answer using animal models. We are now positioned to take what we learn from these patients to guide our studies in the lab and vice versa.
DIMA HAMMOUD, M.D., CC
Senior Investigator and Deputy Director, Center for Infectious Disease Imaging, Radiology and Imaging Sciences, Clinical Center
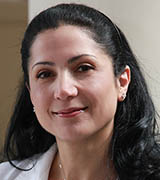
Education: American University of Beirut, Beirut (B.S. in biology; M.D.)
Training: Residency in medicine and in diagnostic radiology at the American University of Beirut–Medical Center (Beirut, Lebanon); fellowships in neuroradiology and in PET imaging at Johns Hopkins Hospital (Baltimore)
Before coming to NIH: Assistant professor of neuroradiology at Johns Hopkins University School of Medicine (Baltimore)
Came to NIH: In 2006 as a staff radiologist; in 2011 became a tenure-track investigator
Outside interests: Painting; building ship models; hiking; cooking; spending time with family
Website: https://irp.nih.gov/pi/dima-hammoud
Research interests: Infectious diseases remain a leading cause of mortality in the world. Molecular imaging, including positron-emission tomography (PET) scanning, can provide valuable insights into disease mechanisms in a noninvasive manner that is very difficult to replicate with other methods. In my lab, we use molecular imaging to better understand the pathophysiology of infection and inflammation by developing novel ligands and testing existing PET probes in animal models of several major infections. The ultimate goal is to translate what is learned into clinical practice.
We have already used those imaging modalities to delineate changes occurring in the brain in the early stages of HIV, including neuronal loss and microglial infection, as well as changes occurring upon starting and stopping antiretroviral therapy. Using PET imaging, we also demonstrated that although HIV-specific changes are still seen in the brains of optimally treated HIV-infected subjects, cognitive dysfunction is probably also due to comorbidities, especially cardiovascular disease.
Our other major interest is developing and validating fungal-specific imaging ligands in animal models. We are exploiting metabolic pathways and cellular constituents that are selectively present in fungi but not in mammalian cells or bacteria. We have already identified multiple promising PET ligands that we are now testing in animal models of fungal infection. We hope we can eventually apply what we’ve learned to patients.
Finally, we are also using molecular imaging to understand high-consequence viral infections and how they affect the brain and other major organs. This work includes evaluation of Ebola-infected monkeys and small-animal models of SARS-CoV-2 disease pathology.
ZAYD M. KHALIQ, PH.D., NINDS
Senior Investigator, Cellular Neurophysiology Section, National Institute of Neurological Disorders and Stroke
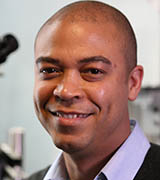
Education: Dartmouth College, Hanover, New Hampshire (B.A. physics); Northwestern University, Evanston, Illinois (Ph.D. in neuroscience)
Training: Postdoctoral fellowship at Harvard Medical School (Boston)
Came to NIH: In 2011 as an Earl Stadtman Investigator
Outside interests: Spending time with his family; running; listening to music; watching documentary films; playing Bach pieces for solo violin
Website: https://irp.nih.gov/pi/zayd-khaliq
Research interests: I am interested in the dopamine system and its contributions to reward and motor learning. Dysfunction of dopamine-releasing neurons in the midbrain has been linked to a variety of brain disorders including addiction, schizophrenia, depression, and Parkinson disease. Although early work established that dopaminergic neurons respond robustly to unexpected rewards, recent studies have revealed that these neurons are engaged by a broader range of behaviors—including novelty and aversion—suggesting that multiple dopaminergic circuits exist. My lab has focused primarily on defining distinct dopamine subcircuits and determining how they contribute to incentivized learning.
One direction in the lab focuses on how dopaminergic neurons located in the substantia nigra (a region of the midbrain) are controlled by projections from the basal ganglia (clusters of neurons in the brain with several functions, including movement). In particular, dopamine neurons are innervated by the dorsal striatum, which is a structure involved in an animal’s choice of future movements. This structure relies on reciprocal input from dopaminergic neurons, which enable movement; the lack of dopamine release in the dorsal striatum leads to Parkinsonism. Although the dorsal striatum is believed to quiet the activity of dopamine neurons, a recent circuit-mapping study from our lab identified a striato-nigral circuit in which striatal input can lead to an increase in activity in a subset of dopaminergic neurons that enhance dopamine release in the striatum [bioRxiv 2020; DOI:10.1101/856617 (preprint server for biology)].
We believe that this dopamine circuit may be a useful way to signal relief from an unpleasant stimulus and may reinforce escape behaviors.
I am also interested in how motor-learning circuits control dopamine release by directly targeting dopaminergic neuron axons. Substantia nigra dopaminergic neurons have axons that branch extensively compared with other central neurons, a feature that increases their energetic cost and may factor in their susceptibility to neurodegeneration.
In a recent study, our lab provided the first direct recordings from the thin dopaminergic axon processes within the striatum [bioRxiv 2020; DOI:10.1101/2020.02.09.941179 (preprint server for biology)]. We hope that these studies will provide important mechanistic insights underlying the diverse behaviors that rely on dopamine signaling such as reward and motor learning and will help us to identify therapeutic targets for disorders—such as addiction and Parkinson disease—that compromise the dopamine system.
JAMES KOCHENDERFER, M.D., NCI-CCR
Senior Investigator, Surgery Branch, Center for Cancer Research, National Cancer Institute
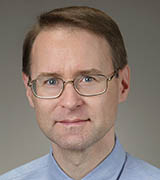
Education: West Virginia University, Morgantown, West Virginia (B.A. in chemistry); West Virginia University School of Medicine, Morgantown, West Virginia (M.D.)
Training: Clinical training in internal medicine at Vanderbilt University (Nashville); oncology fellowship at the University of Texas M.D. Anderson Cancer Center (Houston) and hematology fellowship at Baylor College of Medicine (Houston); further training in tumor immunology and stem-cell transplantation as a clinical fellow at NCI
Came to NIH: In 2002 for training; in 2010, became an assistant clinical investigator; in 2013, became a tenure-track investigator
Outside interests: Exercising; spending time with his family
Website: https://irp.nih.gov/pi/james-kochenderfer
Research interests: My team and I develop T-cell therapies for blood cancers including lymphoma, leukemia, and multiple myeloma. T cells normally play a critical role in fighting infections and cancers. Patients who have cancer have T cells that are not effectively fighting their cancer. With gene therapy, their T cells can be modified to attack a cancer target and exert a powerful and specifically targeted anticancer effect.
My lab genetically engineers T cells with genes encoding chimeric antigen receptors (CAR) that target malignancy-associated antigens. When I was a postdoctoral fellow in Steven Rosenberg’s lab, I designed and constructed a novel anti–cluster of differentiation (CD19) CAR that led to the first FDA-approved CAR T-cell therapy for lymphoma.
We also designed the first chimeric antigen receptor targeting B-cell maturation antigen (BCMA). I led the first clinical trial of T cells expressing an anti-BCMA CAR as a treatment for multiple myeloma. My group has done extensive work with anti-BCMA CARs, including playing a central role in the first multicenter trial of anti-BCMA CAR T cells for multiple myeloma (N Engl J Med 380:1726–1737, 2019; DOI:10.1056/NEJMoa1817226).
We have ongoing clinical trials investigating novel CAR T-cell therapies in multiple myeloma and lymphoma. We are also developing new methods to improve the cancer-fighting ability of CAR T cells.
A barrier to widespread use of CAR T-cell therapy is toxicity, primarily cytokine-release syndrome (CRS) and neurologic toxicity. Manifestations of CRS include fevers, hypotension, hypoxia, end organ dysfunction, cytopenias (reduction in the number of mature blood cells), coagulopathy, and hemophagocytic lymphohistiocytosis (a condition in which there are too many activated immune cells). Neurologic toxicities are diverse and include encephalopathy, cognitive defects, dysphasias (impairments in producing and understanding language), seizures, and cerebral edema. In a recent paper, we reported a new anti-CD19 CAR that was shown to be less toxic than prior anti-CD19 CARs already in clinical use. (Nat Med 26:270–280, 2020; DOI:10.1038/s41591-019-0737-3)
JADRANKA LONCAREK, PH.D. NCI-CCR
Senior Investigator and Head, Centrosome Biology Section, Laboratory of Protein Dynamics and Signaling, Center for Cancer Research, National Cancer Institute
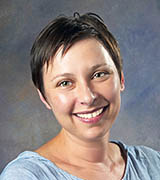
Education: Faculty of Sciences at Zagreb University, Zagreb, Croatia (B.S. in molecular biology; M.S and Ph.D. in cell and molecular biology)
Training: Postdoctoral training at Wadsworth Center, New York State Department of Health (Albany, New York)
Came to NIH: In August 2011 as an Earl Stadtman Investigator
Outside interests: Reading; watching movies; gardening
Website: https://irp.nih.gov/pi/jadranka-loncarek
Research interests: Abnormalities of centrosomes are the hallmark of most tumors, especially aggressive ones. Understanding the molecular mechanisms that regulate centrosome number, structure, and function is paramount for understanding centrosome-related diseases.
A typical dividing human cell has two centrosomes, organelles that organize bipolar mitotic spindle poles and facilitate the segregation of chromosomes during cell division. In the heart of each centrosome is the centriole, a structure that organizes a proteinaceous matrix into a centrosome. The number of centrosomes depends on the number of centrioles.
Aberrant centriole amplification, which destabilizes chromosomes, correlates with a loss of tumor-suppressor proteins, the triggering of oncogenic-virus infections, and DNA damage. Because we lack basic knowledge about how the centriole assembly is regulated during the normal cell cycle, we don’t yet understand the molecular mechanisms that promote centriole amplification.
My lab aims to unravel cellular regulatory pathways that control centrosome formation in normal and pathological conditions such as human tumors. We use biochemical and genetic approaches in combination with conventional, super-resolution, and electron microscopy in our investigations.
In our latest study, we used expansion microscopy (a recently pioneered imaging approach) in combination with electron and super-resolution microscopy to explore the mechanisms that lead to the formation of structurally aberrant centrioles. We discovered that centrioles do not have an elongation-monitoring mechanism. The lack of this mechanism makes them prone to over-elongation in some situations. To the best of our knowledge, ours was the first report showing how centriole over-elongation can occur in cells without chemical or genetic manipulation of centrosomal proteins. Our data can explain the origin of centriole structural variability within cell populations and demonstrates why numerical and structural centriole abnormalities often coincide in tumors. (J Cell Biol 219:e201910019, 2020; DOI:10.1083/jcb.201910019)
WEI LU, PH.D., NINDS
Senior Investigator, Synapse and Neural Circuit Section, National Institute of Neurological Disorders and Stroke
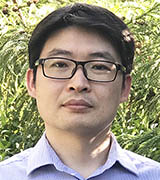
Education: Sichuan University, Chengdu, China (B.S. in biochemistry); New York University, New York (Ph.D. in neuroscience and physiology)
Training: Postdoctoral training in neuroscience at the University of California at San Francisco (San Francisco)
Came to NIH: In 2012 as an Earl Stadtman Investigator
Outside interests: Traveling; playing weiqi (go); engaging in family-oriented activities
Website: https://irp.nih.gov/pi/wei-lu
Research interests: My lab is exploring how the synaptic connections between neurons are formed, how synaptic strength is regulated, what the role of neuronal activity is in the regulation of synapse formation and synaptic plasticity, how the regulation of synaptic strength influences animal behavior, and what the molecular mechanisms underlying synaptic dysfunctions in brain disorders are.
We mainly rely on the rodent hippocampus as our model system for our work. Currently we use molecular, biochemical, and genomic approaches to identify novel players in synaptic function; molecular, genetic, optical, and pharmacological approaches to manipulate synapses; and electrophysiological, genetic, and behavioral approaches to examine synaptic and neural-circuit function and dysfunction.
In a recent study, we found that an interesting gene known as Shisa7 plays a critical role in the regulation of inhibitory neural circuits and the sedative effects some benzodiazepines have on circuit activity. Before this study, it was thought that benzodiazepines, which are powerful sedatives, worked alone to calm nerves. But we discovered that the drugs and the neural circuits they affect need the assistance of Shisa7. We hope the results will help researchers design more-effective treatments for a variety of neurological and neuropsychiatric disorders that are caused by problems with these neural circuits. (Science 366:246–250, 2019; DOI:10.1126/science.aax5719)
NEHAL MEHTA, M.D., M.S.C.E., F.A.H.A., NHLBI
Lasker Senior Investigator and Head, Section of Inflammation and Cardiometabolic Diseases, National Heart, Lung, and Blood Institute
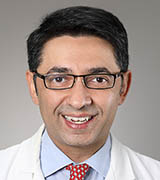
Education: George Washington University seven-year accelerated biomedical program, Washington, D.C. (B.A. in neuropsychobiology; M.D.); University of Pennsylvania, Philadelphia (M.S.C.E. in genetic epidemiology)
Training: University of Pennsylvania: residency and chief residency in internal medicine; fellowship in cardiovascular diseases, nuclear cardiology, and preventive cardiology; postdoctoral fellowship in genetic epidemiology with a focus on human translational studies of inflammation and lipoproteins
Before coming to NIH: Assistant professor, University of Pennsylvania’s Perelman School of Medicine; attending cardiologist, Hospital of the University of Pennsylvania; director, Inflammatory Risk in Preventive Cardiology, Hospital of the University of Pennsylvania
Came to NIH: In 2012 as the inaugural Lasker Clinical Research Scholar
Outside interests: Spending time with his wife and their two daughters; cooking; skiing; surfing; traveling
Website: https://irp.nih.gov/pi/nehal-mehta
Research interests: My research focuses on the role of inflammation in the development of cardiovascular and metabolic diseases. Using a transdisciplinary approach that involves human translational studies, novel cardiovascular-imaging approaches, and a diverse set of applied laboratory-based techniques, my team and I study how inflammation affects the development of insulin resistance, metabolic syndrome, and lipoprotein dysfunction, all of which are factors for developing subclinical atherosclerosis and subsequent cardiovascular events.
I first became interested in this line of research after learning of seminal studies demonstrating that inducing acute inflammation through exposure to lipopolysaccharide could cause insulin resistance in otherwise healthy people. To better understand the mechanism, I used the chronic inflammatory state observed in psoriasis, a common inflammatory skin disease that visibly manifests as thick, scaly plaques, to study the development of cardiometabolic diseases over time. I direct the world’s first and largest study on the effect of psoriasis on cardiometabolic diseases. For more than five years, we have followed over 300 patients who have undergone whole-body cardiovascular imaging as well as flow cytometry for blood. This work has allowed us to do serial evaluation of atherosclerosis over time in a population with high-grade immune and lipid phenotyping.
Research from my lab has demonstrated that people with psoriasis display abnormal lipoprotein particle composition and impairment in high-density lipoprotein efflux capacity, a measure of reverse cholesterol transport. We have also used positron emission tomography to demonstrate that patients with psoriasis have early onset of vascular disease and systemic inflammation, an observation confirmed by our coronary-imaging program using coronary computed-tomography angiography. We also recently described the important role of myeloid cells, namely neutrophils, in the development of atherosclerosis in psoriasis.
Finally, my lab provided the first-in-human proof of concept that treating psoriasis with biologic therapy reduces coronary-artery inflammation and improves coronary-artery disease over time. The effect was of similar magnitude that statin medications provide on coronary plaque! To better understand this observation, we now have studies ongoing in Sweden, Spain, and Australia to assess how new biologic therapy for psoriasis affects coronary disease and cardiovascular events in other populations.
JORDAN MEIER, PH.D., NCI-CCR
Senior Investigator and Head, Epigenetics and Metabolism Section, Chemical Biology Laboratory, Center for Cancer Research, National Cancer Institute
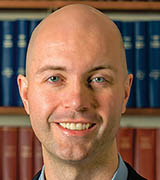
Education: Creighton University, Omaha, Nebraska (B.S. in chemistry); University of California at San Diego, San Diego, California (Ph.D. in chemistry)
Training: Postdoctoral fellow at the California Institute of Technology (Pasadena, California)
Came to NIH: In 2013
Outside interests: Playing and watching basketball; drinking coffee; hoisting children over streams
Website: https://irp.nih.gov/pi/jordan-meier
Research interests: My laboratory aims to understand how metabolites influence epigenetic signaling pathways involved in the development, progression, and treatment of cancer. Metabolites have long been known as building blocks and energetic precursors. In the past decade enormous evidence has demonstrated that metabolites can serve as critical drivers of cellular adaptation. In particular, they have been found to physically interact with enzymes that establish epigenetic protein and nucleic-acid modifications crucial to gene expression. These metabolite-protein interactions occur in all living organisms and represent an essential interface between chemistry and our biological code.
Our lab has defined this interface through a distinctly chemical approach. We have developed proteomic technologies to characterize how metabolites physically interact with enzymes on a proteome-wide scale. With the aid of these “chemical proteomic” methods, we have discovered new protein-metabolite interactions that link metabolism to protein synthesis and also contribute to hereditary kidney cancer. In addition, we have developed technologies—such as nonenzymatic malonylation and RNA cytidine acetylation—for defining the landscape of novel epigenetic modifications. Our overall goal is to better explain the underlying logic linking gene expression and metabolism and to apply this knowledge toward new approaches to cancer therapy, diagnosis, and chemoprevention.
ANANT PAREKH, D.PHIL., NIEHS
Deputy Chief of the Signal Transduction Laboratory and Head of the Calcium Signaling in Health and Disease Group, National Institute of Environmental Health Sciences
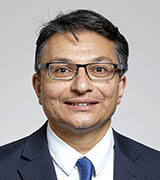
Education: University of Oxford, Oxford, England (D. Phil. in pharmacology)
Training: Postdoctoral fellowship at the Max Planck Institute for Biophysical Chemistry (Goettingen, Germany) with Nobel Laureate Erwin Neher (discoveries concerning the function of single ion channels in cells)
Before coming to NIH: Professor of physiology, director of the Centre of Integrative Physiology, and fellow of Merton College, University of Oxford
Came to NIH: In December 2019
Outside interests: Listening to classical music (particularly Wagner); reading English literature; gardening; playing squash and cricket
Website: https://www.niehs.nih.gov/research/atniehs/labs/stl/pi/calcium_signaling/index.cfm
Research interests: Ever since I attended a physiology lecture in medical school at Oxford, I’ve been fascinated by the underlying mechanisms of how different biological systems communicate with one another. Communication between and within cells is essential for the development and survival of any complex organism. In particular, I am interested in intracellular calcium signaling, which plays a fundamental role inside cells as a universal regulator of cell function. The calcium ion activates many cellular responses including fertilization, neurotransmitter release, muscle contraction, energy production, cell growth, and cell death.
My group at NIEHS studies how aberrant calcium signals may contribute to disease in humans, particularly allergies and asthma. We are using various cell model systems and human tissue to study store-operated calcium-channel proteins. Store-operated calcium channels are so called because they are activated by physiological or pharmacological processes that deplete calcium from bones and other places where it is stored in the body. Once opened, calcium enters the cell and triggers important physiological responses.
Previously, my group at Oxford demonstrated that store-operated calcium channels in the plasma membrane were central to immune-cell activation by specific allergens from the house dust mite.
Drugs that target calcium channels show efficacy in various animal models of asthma. My hope is that by using these compounds to control the channels, we might find an effective means of reducing the severity of airborne allergens and pollutants that damage the lungs.
ARMIN RAZNAHAN, M.D., PH.D., NIMH
Senior Investigator and Chief, Section on Developmental Neurogenomics, Human Genetics Branch, National Institute of Mental Health
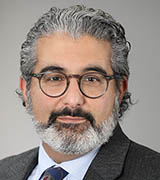
Education: King’s College University London, London (M.D.; Ph.D. in biological psychiatry)
Training: Residencies in pediatrics and psychiatry, and a specialty fellowship in child and adolescent psychiatry at the Maudsley Hospital (London, England); postdoctoral training at NIMH
Came to NIH: In 2008 for a year as visiting Ph.D. student; in 2010 returned as a postdoc; in 2012 became a staff scientist; in 2014 became a NIH-Lasker Clinical Research Scholar
Outside interests: Spending time with his wife and daughter; practicing tai chi; cooking; listening to music; honing the “Dad Joke”
Website: https://irp.nih.gov/pi/armin-raznahan
Research interests: I am interested in achieving a better understanding of the biology of childhood-onset neuropsychiatric disorders in ways that might ultimately help to improve disease prediction, detection, and treatment. Together with our collaborators, my team and I work toward this goal in two mutually informative ways.
First, we use in vivo neuroimaging to study the architecture of human brain organization in healthy volunteers. We also link in vivo neuroimaging maps to complementary molecular and cellular surveys of the human brain. By modeling how human brain organization varies with age, sex, and behavior in health, we hope to advance basic developmental neuroscience while also refining how we ask questions about atypical development.
Second, we use a genetics-first clinical-research strategy to study the relationship between atypical brain development and neuropsychiatric symptoms. This effort involves gathering deep-phenotypic data (spanning measures of gene expression, brain structure and function, cognition, and behavior) in diverse genetic disorders that increase the risk for neuropsychiatric impairment.
These two main research themes are closely interwoven at theoretical, methodological, and empirical levels. For example, we recently developed new ways of linking in vivo neuroanatomical and post-mortem gene expression data in healthy groups (Science, 360:1222–1227, 2018; DOI:10.1126/science.aar25780; Neuron 97:231–247, 2018; DOI:10.1016/j.neuron.2017.11.039). We then used these approaches to show that regional neuroanatomical changes in neurogenetic disorders can be partly explained by intrinsic cell-type patterning of the human brain (Nat Commun 11:article number 3358, 2020; DOI:10.1038/s41467-020-17051-5).
This page was last updated on Tuesday, March 22, 2022