Translating Genetic Findings Into Dementia Treatments
Tracing the Path From Bench to Bedside
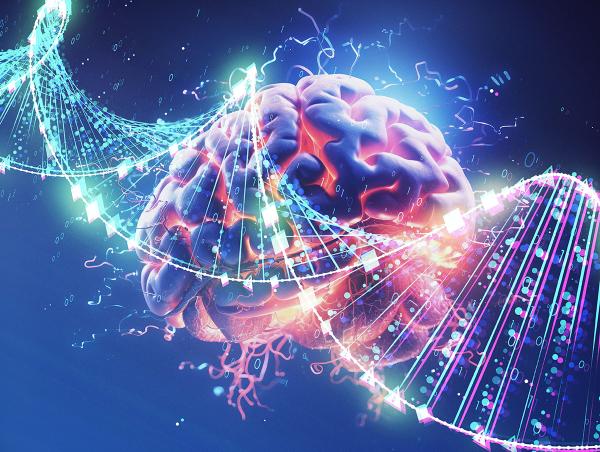
Researchers at NIH’s Center for Alzheimer’s and Related Dementias (CARD) are trying to connect the dots between changes in DNA and the death of neurons in the brain.
When IRP graduate student Pilar Alvarez Jerez looked at the results of a recent experiment, she noticed that when a particular genetic variant is present in a gene called GBA1, it causes a change in the gene's activity. The GBA1 variant, which is associated with Parkinson’s disease and Lewy body dementia, was discovered last year in people of African ancestry by researchers at NIH’s Center for Alzheimer's and Related Dementias (CARD). It appears to suppress the gene’s ability to make a functional version of an enzyme that helps brain cells recycle their proteins.
“This was an interesting finding, but it still didn’t answer how the variant was functioning to lower enzyme activity,” Pilar says.
Pilar and her CARD colleagues were determined to answer that question, employing an array of ‘functional genomics’ techniques to do so. But what exactly is functional genomics, and how does it help translate basic research findings into useful medical treatments?
City Plans — The Genetics of Dementia
It all starts with DNA. In the 1980s and 1990s, researchers identified two genes that produce a pair of key proteins involved in Alzheimer’s disease and found that dementia often runs in families. This led to an explosion of studies into the genetic risk factors underlying the disease.
Over 80 regions within the human genome are now linked to Alzheimer’s, with most increasing risk for the disease rather than directly causing it. For some of these genomic regions, researchers know the exact variant responsible for the link to the disease, which is like knowing the specific address of an apartment. For others, researchers have the general location — like a zip code or the address of an apartment building — but have yet to identify the specific variant.
If the brain is a busy city, the genome is like the city plan. Flaws in the city plan can result in many issues, from dead-end streets and traffic jams to housing shortages. Similarly, some changes in the genome can lead to abnormal, excessive, or insufficient production of important proteins, raising the risk of a disease. Both city planners and dementia researchers must identify problematic planning flaws in order to understand how to fix the problems they cause.
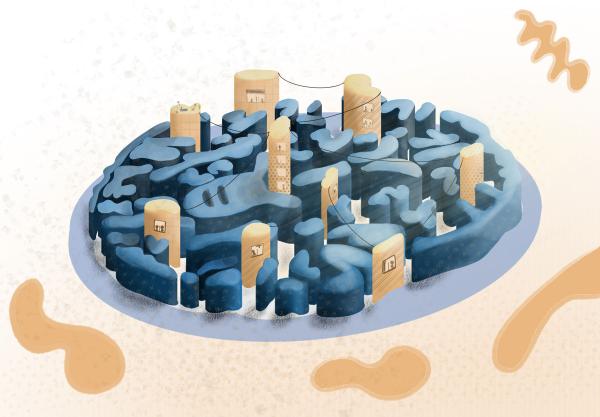
Just like problems with a city plan can lead to traffic jams and other disruptions in a bustling city, genetic mutations can have unanticipated effects on the brain. Illustration by Paige Jarreau
CARD researchers use various techniques to identify novel genetic variants linked to Alzheimer’s. One approach employs genome-wide association studies (GWAS), which compare the genomes of people with and without the disease. Alongside these studies, CARD researchers also employ long-read DNA sequencing, a technique that reliably sequences tricky, repetitive segments of DNA, allowing researchers to identify disease-associated genetic variants that GWAS miss.
It was through a GWAS that Pilar and her CARD colleagues found the novel GBA1 variant that Pilar is studying. They also discovered that the variant interferes with GBA1’s ability to create a protein essential to the function of lysosomes, miniature recycling plants in our cells. However, unlike other GBA1 variants associated with neurological conditions, this novel variant lies in a ‘non-coding’ region of the gene known as an intron. This was curious — how could changing part of a gene that doesn’t directly produce a protein affect the creation of that protein?
Non-Coding DNA — A Genetic Puzzle
Some problems in a city plan are easy to see, like roads that are too close together or too many one-way streets, but sometimes issues with a city plan aren’t immediately visible. For example, all the roads in an area might have weight limits that prevent trucks from delivering goods to that area, but that isn’t readily apparent by just looking at a map.
You can think of changes to a gene’s introns in the same way. Rather than causing obvious changes to the instructions for producing a protein, these regions may regulate the ‘expression’ of genes, altering how they are used to create RNA and how that RNA, in turn, creates a protein. Because introns don’t contain the actual instructions for producing proteins, they need to be cut, or ‘spliced,’ out of the RNA created from a gene before the gene’s protein-making instructions can be carried out. This might make it seem like introns aren’t important, but in fact, GWAS have revealed that most disease-associated genetic variants across all diseases, including Alzheimer’s and other forms of dementia, are in non-coding regions of the genome.
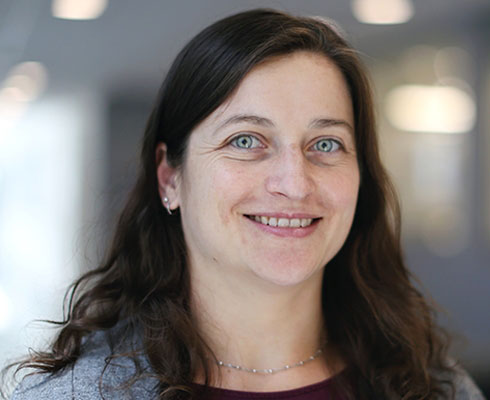
IRP staff scientist Xylena Reed is among the CARD researchers trying to link variation in non-coding regions of our DNA to dementia. Photo by Paige Jarreau
“Around 99 percent of genetic variants are in non-coding regions, either in introns or in the ‘intergenic’ space between genes,” says IRP staff scientist Xylena Reed, Ph.D., a scientist at CARD who leads the Single Cell Sequencing Expert Group. “A variant in an intron could affect the splicing of a gene. It could also regulate a distant gene because the genome folds on itself in complex ways.”
As a result, connecting a non-coding variant to the gene it impacts can be an educated guessing game. Dr. Reed and her colleagues at CARD are working to improve the odds by creating a brain cell atlas of gene regulation and expression. This works like a transparent sheet laid on top of a city plan that shows hidden factors like the weight limits of all the roads. The brain cell atlas includes information about both non-coding segments of DNA and the RNA ‘transcripts’ produced from active genomic regions. Connecting the DNA data to the so-called ‘transcriptomic’ data can show how a given non-coding region of the genome affects gene activity across different types of brain cells, thereby helping researchers make sense of GWAS data to identify the potential cell-specific function of new variants found in non-coding regions.
From Plans to Action — Functional Genomics of Dementia
Even with complete city plans and hidden information revealed, you can’t foresee all potential issues. Understanding how specific genetic changes alter gene expression and contribute to disease is a real challenge. In the case of GBA1, Pilar and her colleagues needed to determine how a variant in a non-coding region of GBA1 impacted the activity of the enzyme produced by this gene, β-glucocerebrosidase, or GCase for short.
Researchers can start answering such questions by comparing the RNA molecules and proteins found in brain tissue, blood samples, and isolated cells from people with and without a given disease. To uncover the impacts of their newly discovered GBA1 variant, Pilar and her colleagues used publicly available datasets to compare blood and other tissue samples from people carrying the variant and those who did not. They discovered that GBA1 expression was higher in people with the variant, meaning the mutated GBA1 gene was producing more RNA than the more common form of the gene does. However, the activity of the resulting GCase protein was lower.
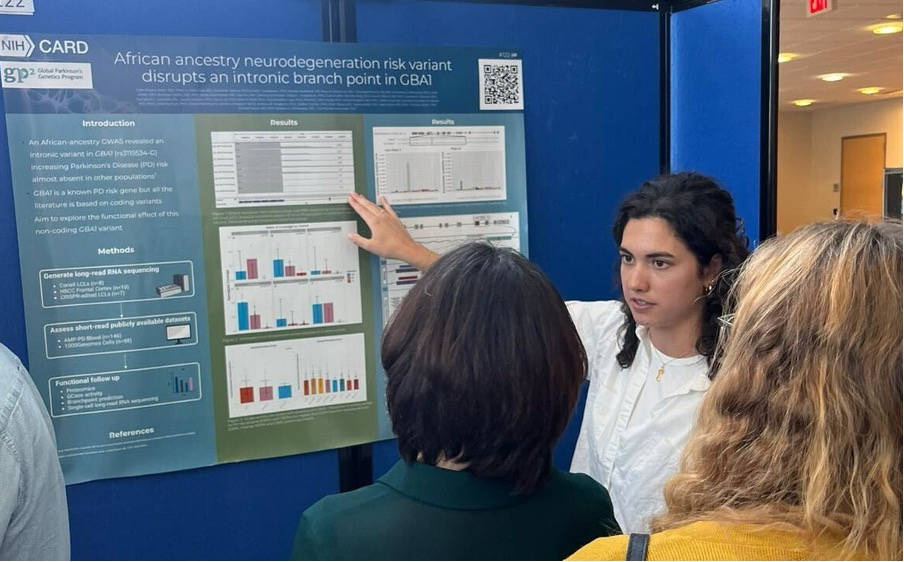
Pilar presents her research on GBA1 at an NIH poster session. Photo courtesy of Pilar Alvarez Jerez
Next, the researchers found some publicly available sets of cells containing the GBA1 variant that were grown from the cells of patients. They grew those cells in the lab and extracted RNA from them, then performed long-read RNA sequencing, which reveals the exact sequence of long sections of RNA. The experiment yielded a curious result. Normally, when DNA is used to create RNA, a gene’s introns will be removed via splicing from the resulting RNA molecule. However, it turned out that part of the intron region where the GBA1 variant lies is not removed from GBA1 RNA.
At first, Pilar and her colleagues thought the abnormal RNA segments might be used to make shortened, non-functional GCase proteins, but they needed to analyze the GCase created by variant-carrying cells to find out for sure. With the help of the CARD Proteomics expert group, they analyzed protein products from cells containing the GBA1 variant, but they found no evidence of abnormal GCase. Rather, they discovered that the abnormal splicing of the GBA1 RNA containing the variant could not be used to make the GCase protein.
Tracking Early RNA and Protein Changes in Dementia
As informative as studying RNA and protein changes in patient samples can be, it doesn’t necessarily answer questions about how a genetic variant triggers these changes in the first place. For example, blood and cell samples derived from patients with dementia are likely to have many changes in various RNA transcripts and proteins associated with the disease that have developed over the course of many years.
“If you arrive in a city in ruins, how do you determine what triggered its downfall?” asks CARD researcher Daniel Ramos, Ph.D.
This is where genetically engineered cell models are helpful, akin to building a miniature of a city based on its plan. Such a model would reveal any early-stage flaws that following the city plan would cause. Researchers working on the iPSC Neurodegenerative Disease Initiative (iNDI) project at CARD are introducing over 100 disease-related genetic variants, one at a time, into induced pluripotent stem cells (iPSCs) derived from disease-free volunteers. These iPSCs are created by manipulating non-stem cells from the volunteers in ways that cause them to revert back to an immature stem cell state. The researchers subsequently coax the iPSCs to mature into disease-relevant cell types like neurons and microglia, then look for abnormal changes in the cells caused by the mutations.
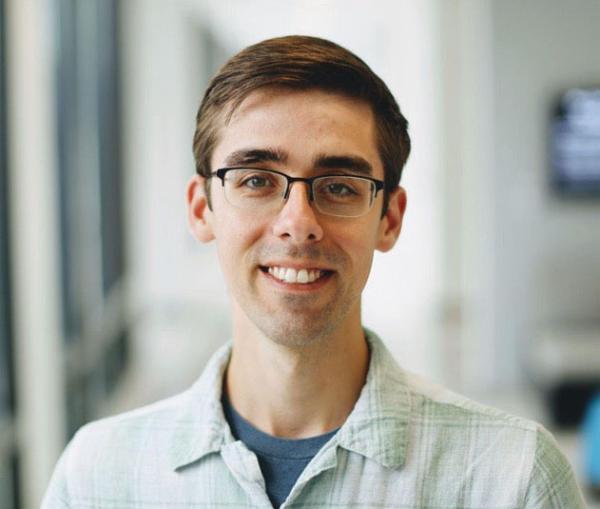
CARD scientist Daniel Ramos. Photo by Paige Jarreau
“We can see how a given genetic variant affects a brain cell's production of RNA and proteins,” Dr. Ramos explains. “We're also looking at many variants in parallel; we’re interested to see if any variants converge and disrupt shared common biological pathways. Some disease-associated variants are likely closely related in what they do, while others have distinct effects, but we're trying to categorize all of them to understand their functions better. This information could lead to a better understanding of these diseases and more robust routes for treatment.”
Pilar and her colleagues used genetically engineered cell lines carrying their novel GBA1 variant to ultimately confirm its mechanism. They introduced their variant into otherwise healthy brain cells and then tracked the resultant changes from beginning to end, through RNA transcripts to proteins.
The team realized they were likely seeing the results of a type of RNA splicing error called a branchpoint disruption. A branchpoint is a site that cell machinery recognizes to initiate splicing. Using a computer algorithm that identifies likely branchpoints in genes, Pilar and her colleagues found that their intronic variant is an important branchpoint in GBA1. When the variant is present, most RNA transcripts produced by GBA1 are abnormal and broken down by the cell before they can be used to create the GCase protein. This leads to less functional GCase and thus lower enzyme activity in the cell.1
“Thanks to CARD's diverse tools and expertise, we were able to use a ‘throw everything at it’ functional genomics approach to solve this puzzle,” Pilar said.
The journey from discovering a genetic variant associated with dementia to developing clinically valuable insights is complex. Through functional genomics techniques, researchers like Pilar, Dr. Reed, and Dr. Ramos are unraveling how genetic mutations contribute to Alzheimer’s disease and related forms of dementia. These efforts enhance our understanding of these diseases and pave the way for more effective treatments.
References:
[1] Álvarez Jerez P, Wild Crea P, Ramos DM, Gustavsson EK, Radefeldt M, Damianov A, Makarious MB, Ojo OO, Billingsley KJ, Malik L, Daida K, Bromberek S, Hu F, Schneider Z, Surapaneni AL, Stadler J, Rizig M, Morris HR, Pantazis CB, Leonard HL, Screven L, Qi YA, Nalls MA, Bandres-Ciga S, Hardy J, Houlden H, Eng C, Burchard EG, Kachuri L, Lin CH, Black DL; Global Parkinson’s Genetics Program (GP2); Singleton AB, Fischer S, Bauer P, Reed X, Ryten M, Beetz C, Ward M, Okubadejo NU, Blauwendraat C. African ancestry neurodegeneration risk variant disrupts an intronic branchpoint in GBA1. Nat Struct Mol Biol. 2024 Dec;31(12):1955-1963. doi: 10.1038/s41594-024-01423-2.
Subscribe to our weekly newsletter to stay up-to-date on the latest breakthroughs in the NIH Intramural Research Program.
Related Blog Posts
This page was last updated on Tuesday, December 17, 2024