Tracking the Neuronal Response to Head Injury
New IRP Study Identifies Vulnerable and Resilient Brain Cells
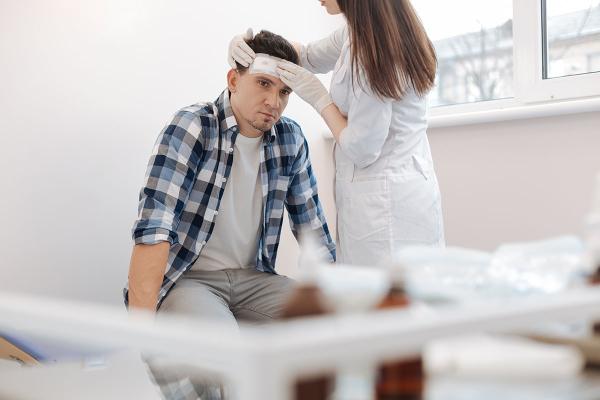
New IRP research sheds light on how different groups of neurons in the brain respond to a head injury.
Even with our thick skulls to protect our fragile brains, a hard enough blow to the head will still send our neurons into a tizzy. However, not all neurons are affected in the same way by a ‘traumatic brain injury,’ or TBI for short. By developing a way to see which neurons turn on a distress signal after a mild TBI, IRP researchers recently identified differences between neurons that die afterwards and those that are shaken up by the injury but manage to recover over time.1
From football players to military personnel, many people experience routine TBIs, and their consequences can add up over time. For instance, repeated TBIs are known to cause the neurological illness known as chronic traumatic encephalopathy (CTE), and they may also increase the risk for developing diseases like Alzheimer’s or Parkinson’s that result from the death of neurons. Yet despite these potentially serious consequences, treatment for a TBI is currently limited to pain management and rest.
“The injury that occurs in TBI is very complex,” says IRP senior investigator Claire Le Pichon, Ph.D., the new study’s senior author. “Many cell types in the brain are damaged and the features of injury can vary widely, so it is difficult to study.”
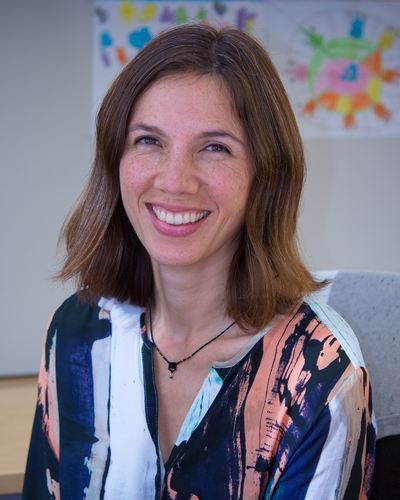
Dr. Claire Le Pichon
Despite not being an expert on TBI, Dr. Le Pichon’s lab decided to tackle the topic in its new study due to its interest in a protein called ATF3. In a prior collaboration between Dr. Le Pichon and IRP senior investigator Nick Ryba, Ph.D., she had generated a mouse model of neuropathic pain in which neurons producing ATF3 can be distinguished from those without the protein. This allowed the scientists to observe that ATF3 becomes more abundant in neurons outside the brain when they are injured and is involved in the cells’ recovery.2 Since other scientists have reported that a TBI similarly causes neurons in the brain to start making ATF3, Dr. Le Pichon wondered if she could use ATF3 to zero in on the specific cells in the brain affected by a TBI and monitor changes in them over time in the same way she had with neurons outside the brain.
“The reason it works well as a signal for neuronal injury is that ATF3 is normally not made in healthy neurons — it’s something that comes on very specifically in neurons that are injured,” she explains.
In the new study, her team tracked neurons that were making ATF3 in a mouse model of mild TBI, which showed that two distinct populations of neurons in the brain’s cortex — its outer layer — started making the protein after a head impact. Intriguingly, the ATF3-producing neurons relatively deeper in the cortex could not be located again two weeks after the injury, suggesting they had died, while the set of ATF3-producing neurons closer to the surface of the cortex were still around 10 weeks after the injury.

The study revealed that neurons in deeper layers of the brain’s cortex seem to be more severely affected by a mild TBI compared to cells in layers of the cortex closer to the brain’s surface.
“From a translational point of view, I think it’s important to know that not all neurons experience the same problems after a TBI,” Dr. Le Pichon says. “If you want to develop a treatment to prevent neurons from dying, it might not be something you want to apply to cells that are resilient to injury. Also, if you learn about the different types of neuronal responses to injury and figure out what’s going on in the neurons that are able to recover, maybe you can apply that knowledge to help the more vulnerable cells.”
And that’s precisely what Dr. Le Pichon’s team decided to do after observing the differing fates of those two sets of neurons. First, the researchers examined the activity of many different genes in both groups of neurons and saw that the more vulnerable neurons deeper in the cortex showed signs of activating a system called the ‘integrated stress response,’ which puts the brakes on the production of new proteins so the cell can recover before restoring normal functioning. Next, the IRP scientists examined the electrical firing of the two sets of ATF3-producing neurons and observed that when they electrically stimulated those vulnerable cells, they did not fire as they normally would. Meanwhile, the more resilient neurons closer to the brain’s surface maintained mostly normal firing; if anything, they fired a bit too much when stimulated.
Finally, the researchers attempted to stop the ATF3-producing neurons deeper in the cortex from dying by manipulating a gene called DLK, which was already known to be turned on in injured neurons and promote their death. When the researchers selectively deleted DLK in those neurons, the cells no longer made ATF3 after a mild TBI and did not die.

Deleting a specific gene, called DLK, prevented the death of neurons after a mild TBI, suggesting a possible therapeutic target for people who are at high risk for such injuries.
“In theory, for people at risk of experiencing a TBI, or if they were about to have a kind of surgery where they might sustain nerve damage, DLK inhibitors might be treatments worth testing as a preventative,” Dr. Le Pichon says. “And if DLK itself turns out not to be a good target, you could think of targeting another player in the same pathway.”
In addition to suggesting the promise of DLK-targeting therapies for helping people at risk of TBI, the study demonstrated the unique insights that come from tracking ATF3-producing neurons over time. Applying the approach to other ailments related to the death of neurons, such as the neurological movement disorder amyotrophic lateral sclerosis (ALS), could yield insights into how to better treat those diseases as well.
“I think we could apply this in a very broad way to potentially any neurodegenerative disease and we’d see that a subset of neurons turns on ATF3, and I think seeing what happens to them could be important to better understanding these diseases,” Dr. Le Pichon says. “You could also imagine specifically targeting that population of neurons to treat these diseases.”
Subscribe to our weekly newsletter to stay up-to-date on the latest breakthroughs in the NIH Intramural Research Program.
References:
[1] Alkaslasi MR, Lloyd EYH, Gable AS, Silberberg H, Yarur HE, Tsai VS, Sohn M, Margolin G, Tejeda HA, Le Pichon CE. The transcriptional response of cortical neurons to concussion reveals divergent fates after injury. Nat Commun. 2025 Jan 27;16(1):1097. doi: 10.1038/s41467-025-56292-0.
[2] Nguyen MQ, Le Pichon CE, Ryba N. Stereotyped transcriptomic transformation of somatosensory neurons in response to injury. Elife. 2019 Oct 8;8:e49679. doi: 10.7554/eLife.49679.
Related Blog Posts
This page was last updated on Tuesday, April 22, 2025