A Year of Honors for IRP Cancer Researchers
Four NIH Scientists Received Prestigious Recognition in 2022
The complexities of cancer, which is actually a collection of many diseases, has made conquering it an enormous challenge. Fortunately, researchers in the NIH Intramural Research Program are up to the task. This year, four IRP investigators in NIH’s National Cancer Institute (NCI) have been recognized for their groundbreaking contributions to answering fundamental questions about the disease and the immune system’s response to similar threats.
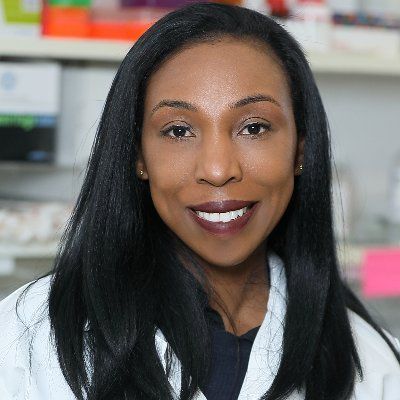
IRP senior investigator Kandice Tanner received the Arthur S. Fleming Award in April.
Kandice Tanner: Tracing Cancer Cells Swimming Through Zebrafish
When you think about cancer research, zebrafish may not be the first thing that comes to mind. Yet these tiny, translucent swimmers are excellent models for studying the disease in humans. That’s why IRP senior investigator Kandice Tanner, Ph.D., harnessed them to conduct her breakthrough research on the way cancer spreads, or ‘metastasizes,’ within the body.
“The site of cancer metastases is not random,” Dr. Tanner says. “Based on where the tumors originate, they show preferential patterns for migration after they’ve left the primary site. There are theories that intrinsic properties of the tumor cells and the target organs must be compatible. It’s like seed and soil. If they mesh well, the new tumor can grow.”
A physicist by training, Dr. Tanner was interested in the factors that cause different types of cancer to spread to certain organs and not others. Her IRP lab uses sophisticated imaging and other measurement techniques to trace skin cancer cells as they move through living zebrafish over time. Those techniques allow Dr. Tanner’s team to measure the physical properties of, and cues from, blood vessels and organs that influence the movement of the cancer cells. In April of this year, her discoveries earned her the Arthur S. Flemming Award, which recognizes exceptional work by federal employees.
Cancer in zebrafish bears a surprising similarity to cancer in humans. This has allowed Dr. Tanner and her team to recreate some aspects of human disease — namely, what happens when tumor cells get stuck in tiny blood vessels called capillaries. This is thought to be one of the precursors to cancer cells spreading to a new organ.
“My background allows me to understand the physical properties of the tissues involved,” says Dr. Tanner, “so I am really focused on trying to identify biomarkers that incorporate physical properties to predict where in the body the tumor will go.”

Some of Dr. Tanner’s experiments involve introducing human tumor cells (magenta) into zebrafish and observing how they spread through the fish’s body. In this image of a five-day-old zebrafish, the fish’s blood vessels are colored green.
Dr. Tanner and her team have discovered that the physical properties of blood vessels themselves regulate the ability of cancer cells to travel to different organs. To spread through the body, tumor cells must recognize certain physical traits of the blood vessels they travel through, such as their size and elasticity, as well as respond to certain chemicals that blood vessels and organs produce. It’s akin to the way a person might use their various senses to help navigate through a busy city, seeking out the sights and sounds of their destination.
Ultimately, Dr. Tanner wants to see the information she has learned from zebrafish put to use in the clinic to predict the onset and progression of human cancer and, eventually, develop treatments. By understanding the early events that guide metastasis, she says, it may be possible to redirect tumor cells in patients to spread to other locations in the body where they are less harmful and easier to treat.
Over the decade that Dr. Tanner has spent at NIH, she has come to believe that the IRP provides fertile ground for someone with her sort of multi-faceted background in both physics and biology. She credits her research team, the resources available at NIH, and the spirit of community-based, multidisciplinary science for her achievements. That’s one reasons, she says, why receiving the Flemming Award was such an honor.
“I think this award is a testament to the type of work that can be done at the NIH,” she says.
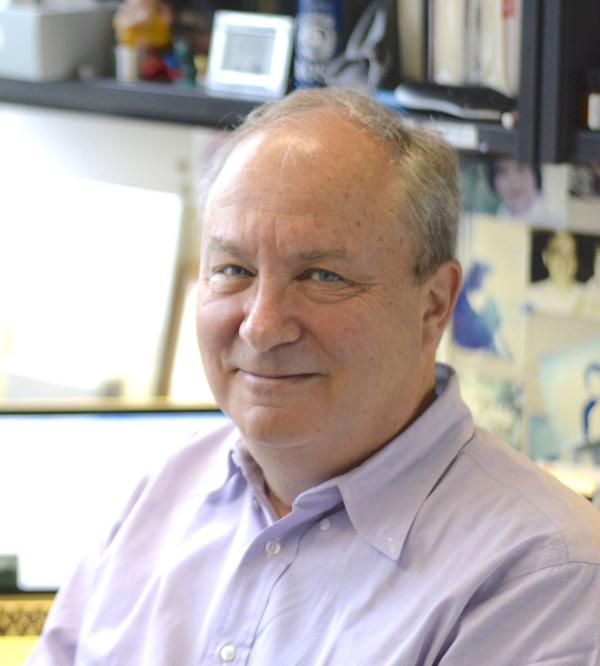
IRP senior investigator Michael Lichten was elected to the National Academy of Sciences in May.
Michael Lichten: Untangling the Mysteries of Cell Division and Repair
Some failures lead to great things. That was the case for IRP senior investigator Michael Lichten, Ph.D., who found he needed a new summer job when his moving business went bust shortly after he graduated from college. Luckily, the Massachusetts Institute of Technology (MIT), where he was already planning to complete his doctoral studies, had room for him in a laboratory studying the way strands of DNA exchange segments in a process called homologous recombination. He went on to do his Ph.D. research on the subject, an experience that set him up to spend the rest of his career unlocking the mysteries of DNA repair, which is fundamental for both avoiding and eliminating cancer.
More than four decades later, in May of this year, Dr. Lichten was elected to the National Academy of Sciences (NAS) for his discoveries about how DNA is damaged and repaired in yeast during meiosis, the process by which a cell replicates its genetic information and then splits into four new cells. Those four newborn cells, known as ‘gametes,’ each have half the amount of DNA of a normal cell, and during sexual reproduction one from each parent must merge together in order to form a fertilized embryo.
“Meiosis is a very useful model for studying DNA damage repair,” Dr. Lichten says. “It has implications for understanding mutations and how to avoid them, particularly in cancer, because so many of our cancer therapies are built on treatments that damage DNA as part of the strategy to kill the cancer cells. Understanding how that damage can be repaired is very important for understanding both how the therapy works and how cancer cells become resistant to those therapies.”
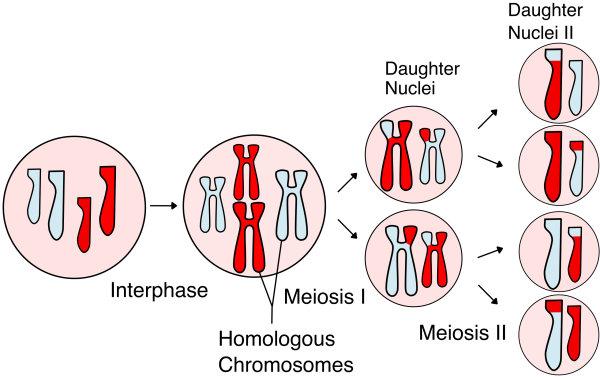
During meiosis, the chromosomes inside a cell exchange genetic material, and the cell ultimately splits into four daughter cells, each with half the original number of chromosomes. Image credit: Rdbickel via Wikimedia Commons
Meiosis naturally involves damage to the DNA as it breaks apart and undergoes the process of homologous recombination that Dr. Lichten first studied at MIT. During recombination, two of the chromosomes DNA is packaged into — one from each of the organisms’ parents — exchange pieces of themselves. In this way, chromosomes swap genetic information to increase the genetic variation in the next generation. One of Dr. Lichten’s most important contributions to our understanding of meiosis was showing for the first time that the types of DNA repair events that happen during meiosis occur via two very different pathways that diverge early in the process. The study that led to that insight was led in the 1990s by a postdoctoral fellow in Dr. Lichten’s lab, Thorsten Allers, Ph.D., now a Professor of Archeal Genetics at the University of Nottingham in the United Kingdom.
“That was revolutionary at the time because people thought recombination occurred by a single pathway that maybe diverged at the end of meiosis,” Dr. Lichten explains.” It changed the way we looked at the mechanism and how these events were regulated.”
Going forward, Dr. Lichten says there’s a lot more work to be done to understand the actual molecular mechanism and proteins that are involved in DNA repair, including finding the critical step where the two different repair pathways diverge. The many researchers Dr. Lichten has trained over the years will be carrying on this work, as he is retiring this year after a long, productive career. That team, he says, all contributed heavily to his success.
“The National Academies honor a career of science, but they don’t honor all the teamwork that goes into it,” says Dr. Lichten of his election to the NAS. “I really do feel that we should be honoring the work first and the person second.”
IRP senior investigator Susan Lea was elected a Fellow of the Royal Society in May.
Susan Lea: Taking Pictures of Invasive Bacteria
Just like cancer cells, bacteria have a range of tricks available to sneak their way through our bodies and infiltrate our organs. Understanding the maneuvers bacteria use when doing battle with our cells is key to developing better ways to arm the immune system not just against bacteria, but potentially against cancer as well.
IRP senior investigator Susan M. Lea, D.Phil, F.Med.Sci., has spent her career using cutting-edge high-resolution imaging techniques to reveal the intricate structural and functional characteristics of dangerous bacteria. The ultimate goal of this work is to locate the parts of them that allow them to interact with our bodies’ cellular receptors and cause diseases such as bacterial meningitis, dysentery, and typhus. Her research is helping to identify how the ways bacteria interact with human cells influence people’s susceptibility to disease.
“We spend quite a lot of time looking at bacteria that live in the blood system or the gut to understand how they bait the immune system and then how they evade it,” Dr. Lea says. “They may try to hide or disguise themselves with stolen bits of our cells or they may carry surface molecules that turn off the immune system. We’re trying to observe these systems directly and understand how an organism causes this.”
In May of this year, two years after Dr. Lea moved most of her lab and several members of her research team to NIH from Oxford University, she was named a fellow of the United Kingdom’s Royal Society, the across-the-pond equivalent of the US’s National Academy of Sciences. Her election as a Royal Society Fellow recognized her pioneering use of structural biology methods to shed light on the fundamental mechanisms behind infectious disease.
“It’s just an incredible honor,” Dr. Lea says of her election. “It's wonderful to have such a recognition of the work — not just my work, but the work of our laboratory group over all these years.”
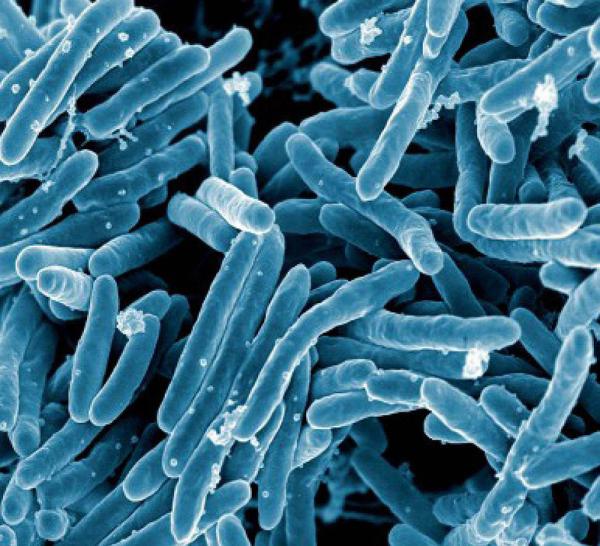
Dr. Lea’s research has produced numerous important insights into the interactions between disease-causing bacteria and our immune systems.
One of the structural mysteries Dr. Lea’s research team recently unlocked was the molecular architecture of the bacterial flagellum, the rotating tail that propels bacteria through their environment like a little outboard motor.
“Even though bacterial flagella have been studied for many years, we actually had very little information about how they are assembled,” Dr. Lea says. “Now we're beginning to really have a molecular understanding of how this little rotary motor works to make bacteria move within and between cells and out in the environment. I think this eventually may lead us toward therapies.”
Indeed, while Dr. Lea’s lab is focused on basic science, her research has clear applications in the clinic. For example, her team worked closely with an Oxford University clinical microbiologist, Christopher Tang, D.Phil, to gain insight into the bacterial molecule that provokes an immune response in a vaccine for meningitis B, a life-threatening bacterial infection affecting the lining that surrounds the brain. They then used the information they learned to re-design and improve the vaccine. In a separate project, in collaboration with researchers in London, Dr. Lea’s team discovered that certain genetic mutations affecting the immune system might make people slightly more resistant to meningitis but also cause their immune systems to overreact to other infectious invaders, leading to autoimmune disease.
“Even though we don’t always set out with a translational question, these pathogen-specific mechanisms often lead to something that actually has direct implications for therapy, or at least an explanation of a disease for patients,” Dr. Lea says. “I think that’s really important.”
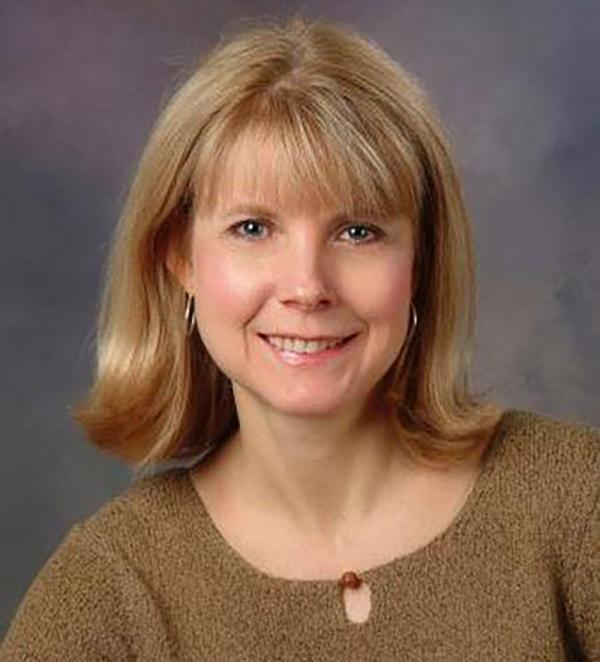
IRP senior investigator Deborah K. Morrison was elected to the National Academy of Sciences in May.
Deborah K. Morrison: Scrutinizing the Structures of Cellular Messengers
In May 2022, IRP senior investigator Deborah K. Morrison, Ph.D., had several reasons to celebrate. A cancer researcher herself, she had just finished her final round of chemotherapy when she returned home to find her inbox overflowing with notes of congratulations. She had just been elected to the National Academy of Sciences (NAS) for her work unlocking the mysteries of cellular signaling pathways and the genetic mutations within them that play a significant role in cancer and certain developmental disorders.
“It was a complete surprise, but it came at a time that was very meaningful for me,” Dr. Morrison says. “Having gone through the first-hand experience of having cancer gave me a new awareness and appreciation for why we do the work we do.”
Dr. Morrison’s research is focused on studying the regulation of proteins involved in signaling pathways that transmit messages to our cells. Cells receive chemical signals passed along from molecules in the body such as growth hormones, and these signals instruct the cells on whether to divide, stay as they are, or die. These signaling molecules bind to receptors located on the outside of cells in order to pass their message inside the cell, where it can be acted upon. Any errors in this chain of events can have serious repercussions, including turning healthy cells into cancerous ones.
Working with the lab of IRP Stadtman investigator Ping Zhang, Ph.D., Dr. Morrison’s team has used a cutting-edge imaging technique called cryogenic electron microscopy to zoom in on two proteins that play a key role in regulating the growth, division, and migration of cells, called RAS and RAF. The RAS protein, found in all animal cells, acts as an on-off switch to drive those cellular functions. RAF, meanwhile, is an enzyme that interacts directly with the RAS protein and is essential for transmitting RAS’ instructions into the cell.
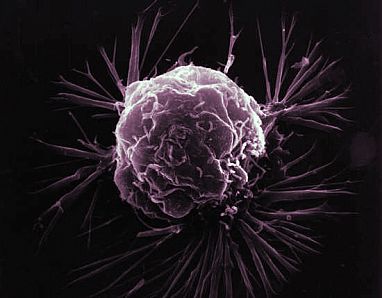
Mutations in the RAS and RAF genes can cause cells to divide uncontrollably, eventually giving rise to cancerous tumors.
While RAS and RAF are essential for life, mutations in the genes that produce them can cause serious health problems. For instance, mutations in a cell’s RAS gene can jam the switch into the ‘on’ position, causing constant and uncontrolled cellular division. This is a hallmark of cancer, and in fact RAS mutations are found in up to 30 percent of tumors. Mutations affecting RAS and RAF can also occur in a developing embryo, causing the proteins to work incorrectly in every cell in an infant’s body and resulting in serious developmental disorders or death.
“Normally this pathway gets turned on and then shut off as needed, but in cancer, this pathway is always turned on,” Dr. Morrison says. “Recently, we determined the structure of the full-length RAF protein and visualized how it interacts with RAS. It is almost like seeing how you can fit Legos together, and then you can see if there are places where you could prevent or disrupt their interactions.”
This structural information and greater understanding of how the RAS signaling pathway is regulated may one day be used to design new strategies for personalized treatments for cancer and developmental disorders. For example, this past year, Dr. Morrison’s research team demonstrated that an important aspect of the signaling pathway is ‘autoinhibition,’ which keeps the RAF protein in a resting state. Some mutations can weaken this autoinhibition, allowing growth signals to be transmitted to the cell when they shouldn’t be. Dr. Morrison’s lab is now using zebrafish as animal models to develop tests that predict whether a particular mutation will cause disease and determine the severity of the mutation’s effects.
“Such information may be very beneficial to both doctors and parents of children with developmental disorders as they think about the types of treatments to pursue,” Dr. Morrison says.
Subscribe to our weekly newsletter to stay up-to-date on the latest breakthroughs in the NIH Intramural Research Program.
Related Blog Posts
This page was last updated on Wednesday, July 5, 2023