Colleagues: Recently Tenured
ROBERT B. BEST, PH.D., NIDDK
Senior Investigator, Theoretical Biophysical Chemistry Section, Laboratory of Chemical Physics, National Institute of Diabetes and Digestive and Kidney Diseases
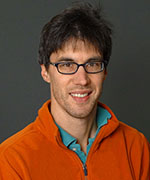
Education: University of Cape Town, Cape Town, South Africa (B.Sc. and M.Sc. in chemistry); University of Cambridge, Cambridge, England (Ph.D. in chemistry)
Training: Postdoctoral training at University of Cambridge; postdoctoral training at NIDDK
Before coming to NIH: Royal Society University Research Fellow, Chemistry Department, University of Cambridge; college lecturer at Emmanuel College, University of Cambridge
Came to NIH: In 2004 for training; in 2012 as an investigator in NIDDK
Selected professional activities: Editorial board member of Scientific Reports
Outside interests: Running; rowing
Website: https://irp.nih.gov/pi/robert-best
Research interests: The Theoretical Biophysical Chemistry Section’s main goal is to develop the theoretical framework needed to understand the dynamics of molecules at the single-molecule level. We use theory and molecular simulations to help to explain and understand experimental observations in terms of the complex microscopic processes involved.
Through the impressive achievements of structural biology, much has been learned about the function of proteins and the structures of their stable states, but it is still challenging to study the dynamics and mechanisms of transitions between these states. Experimental information on dynamics is generally limited in resolution. To aid in its interpretation, my lab is developing simulation and theoretical methods for studying macromolecular dynamics and applying them to biologically interesting systems. In doing so, we have resolved several controversies related to ambiguities of experimental interpretation. In complementary work, we have been using empirical data for peptides and macromolecules in solution to further improve the energy functions used in our simulations.
My lab’s other recent projects include performing experiments using atomic-force microscopy and optical tweezers to study protein folding in the presence of an external “pulling” force. We also resolved a long-standing discrepancy between small-angle X-ray scattering and Förster resonance energy transfer experiments on intrinsically disordered proteins. In addition, we developed coarse-grained master equations as a tool for interpreting peptide dynamics in simulations and used diffusion models of protein folding. My lab also determined the binding mechanism of intrinsically disordered proteins. We studied the influence of molecular chaperonins on folding and misfolding as well as the self-association of transmembrane helices. We also identified the mechanisms of substrate transport in hydrogenase enzymes and developed methods for identifying cryptic binding pockets in proteins.
We hope that a deeper understanding of biological processes and how molecules function may one day lead to discoveries that will contribute to improved treatments for diseases and disorders.
CHOONSIK LEE, PH.D., NCI-DCEG
Senior Investigator and Head of the Dosimetry Unit, Radiation Epidemiology Branch, Division of Cancer Epidemiology and Genetics, National Cancer Institute
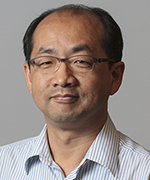
Education: Hanyang University, Seoul, South Korea (B.S.E. in nuclear engineering; M.S. in health physics; Ph.D. in health physics)
Training: Postdoctoral fellow, Innovative Technology Center for Radiation Safety (Seoul); postdoctoral fellow in computational medical physics at the University of Florida (Gainesville, Fla.)
Came to NIH: In 2009
Selected professional activities: Member of the International Commission on Radiological Protection; editorial boards of Radiation Protection Dosimetry Journal and Journal of Radiation Protection
Outside interests: Reading books; listening to classical music; playing the piano and guitar
Websites: https://irp.nih.gov/pi/choonsik-lee; https://ncidose.nci.nih.gov
Research interests: The medical use of radiation has increased dramatically over the past few decades for diagnostic purposes as well as for radiation therapy. For example, computed-tomography (CT) scans performed in the United States increased from 3.6 million in 1980 to 70 million in 2007; approximately 10 percent of them were for pediatric patients, who are at higher risk of radiation-related cancer than adults are. To ensure patient safety, we need a better understanding of how the nature and magnitude of radiation doses used in medical procedures affect human health.
One of the ways we can estimate the doses of ionizing radiation delivered to patients is by using computational human phantoms (computer simulations of the human body) and radiation-exposure scenarios coupled with the Monte Carlo model of radiation-transport techniques. (The Monte Carlo model, named for the international capital of gambling, is a mathematical procedure for generating random numbers and so named because of the element of chance.) Several studies have validated that the simulation approach adequately represents the physical interaction of radiation in the real human body.
I have developed a series of computational human phantoms that are crucial for realistic individualized dose calculations. The International Commission on Radiological Protection has adopted the phantom series as an international reference to ensure the accuracy of individualized organ dosimetry in epidemiological studies of medical radiation and to estimate and monitor the dose given to patients undergoing diagnostic radiation procedures. I am also developing dosimetry methods to calculate and assess the radiation dose received during diagnostic radiation procedures such as CT scans, radiography, interventional fluoroscopically-guided procedures, and nuclear medicine examinations.
Although radiation therapy has been successfully used to treat many types of cancers since the 1920s, there has been an increasing concern about the long-term risk of radiation-induced second cancers among cancer survivors. With external-beam radiation therapy, it is practically impossible to irradiate tumors without some radiation penetrating through the surrounding normal tissues. Many epidemiologic studies of pediatric and adult patients who received radiation treatment have demonstrated late effects including the increased risk of developing second cancers.
However, there are substantial difficulties in reconstructing organ doses for large patient cohorts treated years ago. I am collaborating with extramural clinical medical physicists and oncologists to develop new dose-reconstruction methods in a system called the NCI dosimetry system for radiation treatment (NCIRT). I am working with NCI epidemiologists to apply the tool to multiple epidemiological studies of second cancer in radiotherapy patients. I am also extending NCIRT to include dosimetry methods for proton-therapy patients.
LUIGI DANIELE NOTARANGELO, M.D., NIAID
Senior Scientist, Chief, Immune Deficiency Genetics Section, and Deputy Chief, Laboratory of Host Defenses, National Institute of Allergy and Infectious Diseases
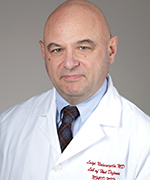
Education: University of Pavia, Pavia, Italy (M.D.)
Training: Residency in pediatrics and subspecialty training in allergy/immunology and human cytogenetics at the University of Pavia; postdoctoral training at NCI’s Metabolism Branch
Before coming to NIAID: Professor and chair of Pediatrics at the University of Brescia (Brescia, Italy; 2000–2006); professor of pediatrics, Boston Children’s Hospital, Harvard Medical School (Boston; 2006–2016)
Came to NIH: In 1986–1988 for training in NCI; joined NIAID in 2016
Selected professional activities: Past president of the European Society for Immune Deficiencies and of the Clinical Immunology Society; chief editor, Frontiers in Immunology
Outside interests: Listening to classical music; playing chess; reading novels; traveling
Website: https://www.niaid.nih.gov/research/luigi-daniele-notarangelo-md
Research interests: My lab studies the molecular and cellular mechanisms of human primary immune deficiencies, especially combined immunodeficiencies (CID), with the ultimate objective of improving the diagnosis and treatment of these conditions. We are collaborating with other NIAID investigators as well as several groups worldwide.
People with CID have an increased susceptibility to infections and often manifest immune dysregulation. We study patients who have genetically undefined forms of CID as well as those with known genetic defects. In the former group of patients, we use whole-exome sequencing and a wide array of cellular and molecular techniques to identify novel gene defects including mutations in DOCK2, TTC7A, HOIP, and EXTL3 that result in decreased concentrations of their proteins. We also have a strong interest in defining the molecular and cellular defects in newborns identified with T-cell lymphopenia.
In patients with CID of known genetic etiology, we are defining the mechanisms underlying immune dysregulation. We are especially interested in human recombination-activating gene (RAG) deficiency. We have determined that the severity of clinical manifestations associated with mutations in the RAG genes correlates with the residual activity of their resulting proteins and with different degrees of perturbation of the T- and B-cell repertoire’s diversity and composition. We are testing the hypothesis that mutations in the coding flank sensitive region of RAG1 may alter the selection of V, D, and J genes targeted for rearrangement, and that skewing of the repertoire may contribute to autoimmunity. These human studies are complemented by similar efforts in novel animal models that we have generated by editing genes with the CRISPR/Cas system.
My laboratory has also developed a large repository of induced pluripotent stem cells (iPSCs) from patients with CID and other immune deficiencies. We aim to define the pathophysiology of immune and extra-immune manifestations of disease. We want to apply CRISPR/Cas technology to correct the gene defect in patient hematopoietic stem cells and iPSCs as a first step toward a future therapeutic use of this strategy.
KUMARAN RAMAMURTHI, PH.D., NCI-CCR
Senior Investigator, Laboratory of Molecular Biology, Center for Cancer Research, National Cancer Institute
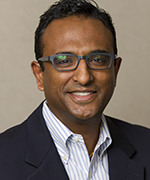
Education: University of California, Los Angeles (B.S. in microbiology and molecular genetics; Ph.D. in molecular biology)
Training: Postdoctoral fellow, Department of Molecular and Cellular Biology (Harvard University, Cambridge, Mass.)
Came to NIH: In 2009
Selected professional activities: Editorial board of the Journal of Biological Chemistry; co-director of the NIH–Johns Hopkins University Graduate Partnership Program
Outside interests: Studying to be a master sommelier
Website: https://irp.nih.gov/pi/kumaran-ramamurthi
Research interests: I am investigating the fundamental mechanisms of cell differentiation and division to determine how they can go awry during disease. My lab focuses on how proteins localize to particular subcellular locations and assemble to form large structures during development and cell division. We discovered, for example, that the shape of cellular membranes, either convex or concave, may recruit certain membrane shape-sensing proteins to their correct destination, a novel mechanism for subcellular protein localization.
A longstanding challenge in developmental biology is understanding how an organism constructs large structures that help define the way it looks. We are approaching this problem by examining the morphogenesis of a simple organism—a bacterial spore. Spores are dormant cells that are encased in a thick protein shell, or “coat,” which helps protect the spore from environmental insults. We are using genetic, biochemical, cytological, and biophysical approaches to investigate how the spore coat is assembled.
We discovered that the assembly of the basement layer of the coat depends on a tiny protein, which anchors the coat onto the surface of the developing spore, and that this protein localizes properly by preferentially embedding in convex membranes such as those found on a spore’s surface. This shape-sensing protein then recruits an unusual cytoskeletal protein that polymerizes by hydrolyzing adenosine triphosphate to form a stable platform upon which other coat proteins eventually assemble. Recently, using defined components, we reconstituted the spore-coat basement-layer assembly in a test tube to construct synthetic spore-like particles that can be modified to display molecules of interest. We are currently testing whether these synthetic bacterial cells may be used as versatile platforms for the display of vaccines and for tissue-specific delivery of drugs.
JIANXIN SHI, PH.D., NCI-DCEG
Senior Investigator, Biostatistics Branch, Division of Cancer Epidemiology and Genetics, National Cancer Institute
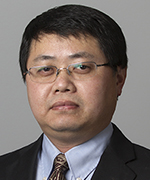
Education: Beijing University, Beijing, China (B.S. in mathematics; B.S. in economics; M.S. in information science); Stanford University, Stanford, Calif. (Ph.D. in statistics)
Training: Postdoctoral fellow, Stanford University School of Medicine
Before coming to NIH: Research scientist in the Health Research and Policy Department and in the Department of Psychiatry and Behavioral Sciences, at Stanford University School of Medicine
Came to NIH: In 2009 as tenure-track investigator in NCI-DCEG
Selected professional activities: Statistics editor for Journal of National Cancer Institute; associate editor for Biostatistics; associate editor forsStatistics in Biosciences
Outside interests: Fishing; listening to classical music
Website: https://irp.nih.gov/pi/jianxin-shi
Research interests: My research focuses on developing and applying quantitative methods—including statistical genetics, tumor sequencing, and human microbiomics—to analyze high-dimensional genomic data in order to understand cancer etiology and improve cancer prevention.
During my postdoctoral fellowship, I worked on genome-wide association studies to identify common genetic variants associated with the risk of developing complex diseases such as breast cancer, schizophrenia, and major depression. Since joining NCI, I have been developing methods for detecting copy-number variations associated with complex disease risk and for identifying genetic variants associated with gene expression and DNA methylation traits.
In addition, I collaborated with two other NCI-DCEG senior investigators on a family-based whole-exome sequencing study to identify a founder mutation in the gene POT1 (which results in the protein Protection of Telomeres 1). I also worked with a scientist at Johns Hopkins University (Baltimore) to develop statistical methods for improving genetic-risk prediction for complex diseases.
Another interesting problem is evaluating the role of genetics in cancer survival. Many studies show that the genomes of tumors influence survival, but researchers do not know whether a patient’s own genome affects survival. I have been developing a method for estimating genetic heritability of survival in lung-cancer patients. Preliminary results showed that that common germline genetic variants do not contribute to the variation of survival in these patients. I plan to expand this work to other cancers.
In another collaboration with one of the NCI-DCEG scientists, we are using samples from the Environment and Genetics in Lung Cancer Etiology study to do an integrative genomic analysis of lung cancer. We are characterizing the intratumor heterogeneity to determine what events drive lung cancer and are identifying genomic features that are predictive of clinical outcomes. We also developed statistical methods to analyze the evolution of tumors.
In addition, I am using large-scale prospective studies to estimate the overall contribution of the oral and gut microbiomes to the risk of developing cancers.
These are some of the recently tenured investigators who will be featured in an upcoming issue of the NIH Catalyst:
- Theo Heller, NIDDK
- Jose Feraldo-Gomez, NHLBI
- Zhiyong Lu, NLM-NCI
- Anil Chaturvedi, NCI-DCEG
- Peter Crompton, NIAID
- Zhengping Zhuang, NCI-CCR
- Joshua Sampson, NCI-DCEG
This page was last updated on Monday, April 11, 2022