Helping Aging Hearts Get Their Groove Back
IRP Researchers Discover ‘Coupled-Clock’ That Controls Heart Rhythms
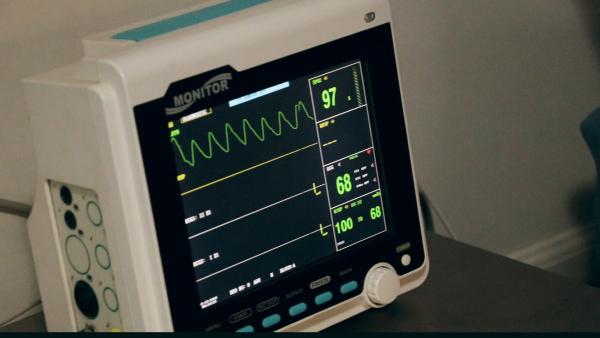
IRP research is revealing new potential strategies for correcting irregular heart rhythms and averting heart failure.
Like so much about our lives, our hearts slow down as we age. While this slowing is natural, a heartbeat that is too sluggish can lead to heart failure, irregular heartbeats known as arrhythmias, and other problems. IRP senior investigator Edward G. Lakatta, M.D., has changed the paradigm in our understanding of how our hearts keep the beat across our life spans — and what happens when they don’t.
When heart rhythms go awry, the most common treatment is implantation of an electrical pacemaker. These devices deliver the electrical charges the heart needs to maintain a steady pace, and as many as 3 million Americans have them, according to some estimates. However, installing a pacemaker is invasive and costly, and as with any surgical procedure, complications can occur. That’s why scientists like Dr. Lakatta are looking for other ways to restart or replace the heart’s natural timekeeper.
When Dr. Lakatta arrived at NIH in 1976, he focused on understanding how the heart’s ventricles work as a pump. The dogma at the time, he says, was that the heart rested between each beat.
“But I started to notice little things happening in between beats,” Dr. Lakatta says. “That’s when I discovered some oscillatory behavior related to local calcium releases. That led me to the discovery of the ‘coupled-clock’ system.”
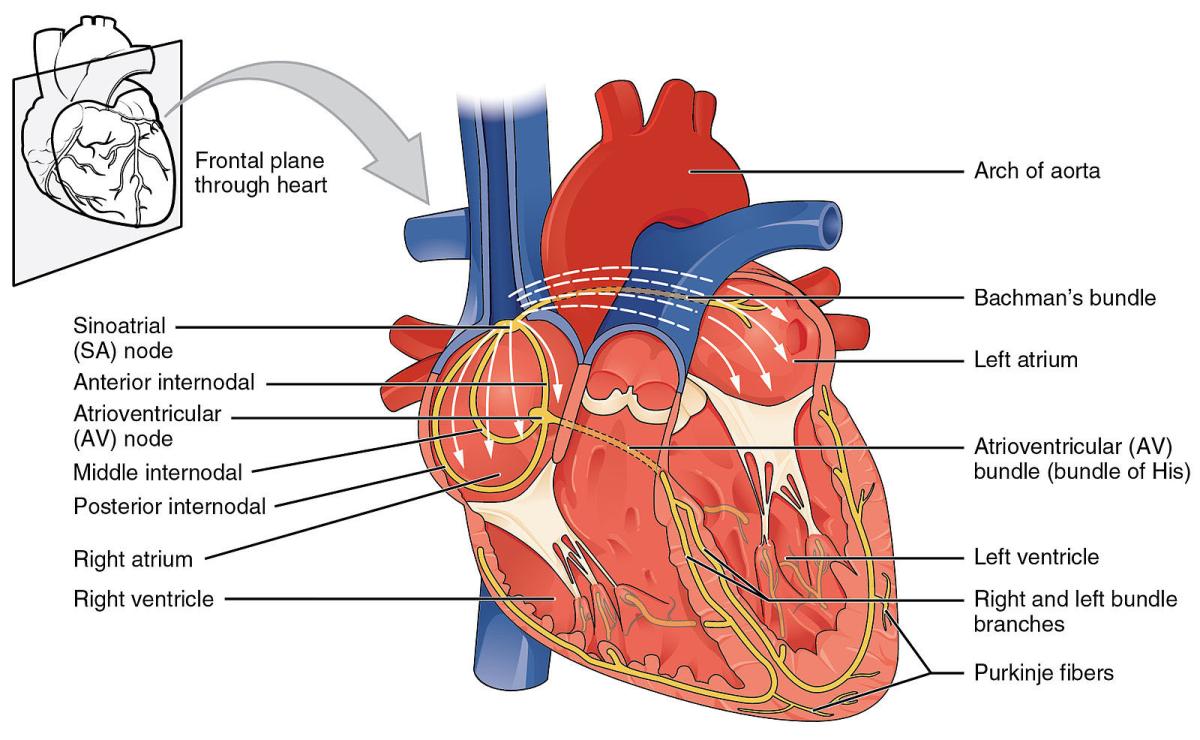
Anatomy of the human heart. Image credit: OpenStax College via Wikimedia Commons
The coupled-clock refers to two timekeepers present in the heart. The ‘calcium clock’ is the regular, timed release of calcium ions by pacemaker cells in the heart’s sinoatrial node, a small body of specialized muscle fibers in the right atrium, one of the heart’s four chambers. These charged calcium particles interact with electrically conductive molecules on the cell membranes of cardiac cells, which Dr. Lakatta and IRP colleagues dubbed the ‘membrane clock.’
The combination of the calcium clock and membrane clock form what Dr. Lakatta’s group calls the coupled-clock system, syncing together to drive normal heart rhythms. However, the two clocks can fall out of sync, and Dr. Lakatta and his team believe that uncoupling could be a potential mechanism for arrhythmias and, in extreme scenarios, heart failure. Consequently, the coupled-clock system may provide a novel therapeutic target for cell-based therapies aimed at restoring a regular, healthy heartbeat, thereby reducing the need for mechanical pacemakers.
In 2018, Dr. Lakatta’s lab moved from experiments in animal models to human tissues and found the same coupled clock at work in our own cells.1 Soon after, the scientists began investigating how the heart’s pacemaker cells know what pace is appropriate for a given situation. In just milliseconds, the central nervous system (CNS) analyzes information like sight, sound, and temperature and directs the heart to beat faster or slower in response. For instance, when we sleep, it will tell the heart to slow down. During exercise, on the other hand, it will send the message to speed up.
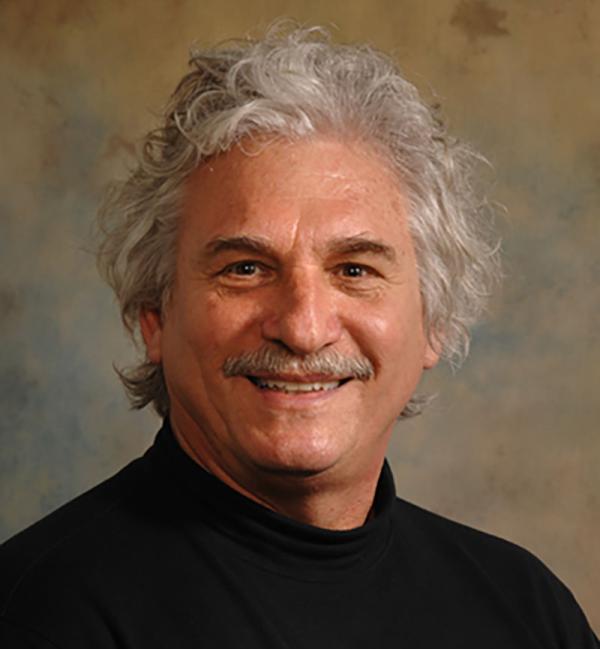
Dr. Edward Lakatta
Using a very high-powered confocal microscope to look at the sinoatrial node in more detail, the IRP scientists discovered that its cellular architecture looks very much like that of the brain.2 Within the sinoatrial node, Dr. Lakatta’s team found cells that communicate with the pacemaker cells in a manner similar to the way neurons send signals in the CNS.
“This observation allowed us to conceptualize the sinoatrial node as a brain within the heart,” Dr. Lakatta says. “The CNS brain sends impulses through its neurons to the heart tissue to communicate messages from your CNS brain to your heart brain.”
Once Dr. Lakatta and his team determined the connection between these two ‘brains,’ they started looking into how aging gums up the works. They knew from findings from the Baltimore Longitudinal Study of Aging that the heartbeat slows by about a beat per year in aging humans. They decided to replicate that observation using a mouse model by comparing the animals’ heart rhythms over time.3 For about the first two-thirds of life, the animals’ heart rates remained consistent, but when the mice reached old age, the time between beats slowed considerably. When the researchers blocked input from the CNS to the heart, that deceleration was even more pronounced. The culprit, Dr. Lakatta and his team determined, was calcium.
“The heart can’t process the calcium signals very fast in old age,” explains Dr. Lakatta. “The frequency of heartbeats is calcium-dependent, but as they age, the pacemaker cells can no longer hold on to the calcium ions, so they can’t retain a sufficient load to generate fast electrical impulses to keep the pace up. You have to wait longer for the next beat and it’s more uncertain when it will come, so the intervals are more variable.”
Dr. Lakatta likens the heartbeat to music; in fact, he even plotted the intervals between heart beats on a musical staff — with faster beats as higher pitches — and then played it on a synthesizer. When he compared the heart songs of mice at different ages, he found a marked difference: the song of the younger mice was a melody, with a greater variety of high and low pitches, while that of the older mice sounded more like a bass line.
“The aged sinoatrial node cannot remember how to hit the high notes — that is, the faster frequency of heart beats,” says Dr. Lakatta. “This is how frailty occurs in the heart. It can’t remember how to ignite the heartbeat. If the heart can't remember how to hit those high notes, it just generates the low ones, and so it has a different rhythm.”
In this video, Dr. Lakatta shows off musical recordings that reflect the heartbeats of the same mouse at age 6 months and 30 months. The recordings also demonstrate how the animal's heartbeats change when the heart lacks 'autonomic input,' meaning the central nervous system is unable to send signals to the heart.
Now, Dr. Lakatta’s team is working to move its discoveries from the bench to the bedside. One approach the group is eyeing is to use external sensory stimuli, such as sound waves and light, to jumpstart sluggish automatic bodily functions like breathing, blood pressure, and heart rate. Dr. Lakatta and his colleagues are putting that idea to the test in a study using exposure to near-infrared light to try to jog the sinoatrial node’s memory of how to beat faster.
“The idea is, if you could break in anywhere into the reflex arc that drives those automatic functions — say through smell, vision, touch, vibrations, or sound — it could have a positive therapeutic effect,” Dr. Lakatta says. “Everything is connected through these automatic reflexes. That’s the way I think organisms were designed, but until now we haven’t really recognized it as a route for therapies. It’s like it’s too good to be true.”
Subscribe to our weekly newsletter to stay up-to-date on the latest breakthroughs in the NIH Intramural Research Program.
References:
[1] Tsutsui K, Monfredi O, Tagirova S, Maltseva L, Bychkov R, Kim M, Ziman B, Tarasov K, Tarasova Y, Zhang J, Wang M, Maltsev A, Brennan J, Efimov IR, Stern M, Maltsev V, Lakatta E. A coupled-clock system drives automaticity of human sinoatrial nodal pacemaker cells. Sci Signaling. 2018; 11(534). doi:10.1126/scisignal.aap7608.
[2] Bychkov R, Juhaszova M, Calvo-Rubio Barrera M, Donald LAH, Coletta C, Shumaker C, Moorman K, Tagirova Sirenko S, Maltsev AV, Sollott SJ, Lakatta EG. The heart’s pacemaker mimics brain cytoarchitecture and function: Novel interstitial cells expose complexity of the SAN. J Am Coll Cardiol EP. 2022 Oct 8; 10:1191–1215. doi.10.1016/j.jacep.2022.07.003.
[3] Moen JM, Morrell CH, Matt MG, Ahmet I, Tagirova S, Davoodi M, Petr M, Charles S, de Cabo R, Yaniv Y, Lakatta EG. Emergence of heartbeat frailty in advanced age I: perspectives from life-long EKG recordings in adult mice. GeroScience. 2022; 44:2801–2830. doi: 10.1007/s11357-022-00605-4.
Related Blog Posts
This page was last updated on Tuesday, May 23, 2023