A New View of How Muscles Move
IRP Research Challenges Long-Held Ideas About Muscle Structure
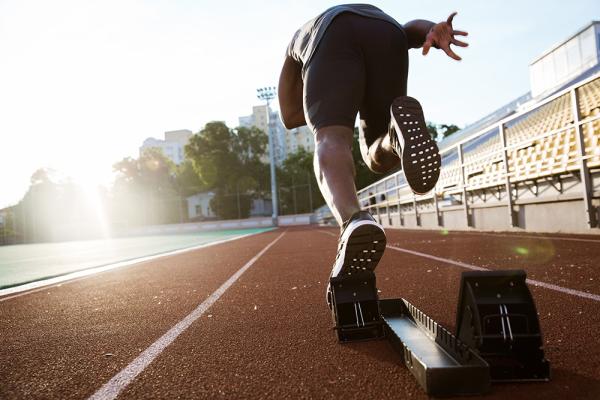
Recent IRP research could overturn long-held views of how our muscles power movement.
It’s not every day an accidental observation overturns 100 years of biological knowledge. But that’s what happened when IRP Stadtman Investigator Brian Glancy, Ph.D., noticed something funny while reviewing high-definition 3D videos of muscle cells.
“To be honest, you could almost call this study an accident,” he says.
Dr. Glancy, who leads the National Heart, Lung, and Blood Institute (NHLBI)’s Muscle Energetics Lab, often uses the high-powered microscopes available through the NHLBI Electron Microscopy Core to study how energy is distributed through skeletal muscle cells — the ones that control voluntary movement — when they expand and contract.
Although he was focused on examining the cells’ energy-producing mitochondria, he could also see the other structures inside them, including the long, tube-like structures called myofibrils that are involved in muscle contraction. As he advanced the video and traveled down the length of the muscle, it looked to him like the myofibrils were changing shape.
“That isn’t what I learned in my exercise physiology and biology courses,” Dr. Glancy remembers. “I basically traced out some of these structures and saw that they were forming branching networks and I thought, ‘That’s weird.’”
For more than a century, scientists thought the myofibrils in muscle cells were made up of long, thin bundles of parallel tubes stretching from one end of the cell to the other. Images of them in textbooks from high school biology to medical school anatomy resembled bundles of fiberoptic cables wrapped in a plastic casing. The force required for muscles to contract, thereby moving our tendons and bones, was thought to travel the length of the tubes.
However, there were some hints that idea might be too simplistic. More than 35 years ago, scientists proposed that contractile force could be transmitted side to side across the width of a muscle cell, in addition to along its length. Yet without the technology needed to adequately study the structure of myofibrils, how exactly those forces spread laterally remained unclear.
That changed with Dr. Glancy’s discovery, published in 2020.1 Using images of cells from mice, Dr. Glancy and his lab showed that myofibrils are actually not made of individual pieces lying side by side. Instead, they actually have little connections where the tubes branch off or split and connect to adjacent ones. Rather than a set of parallel lanes on a straight stretch of highway, it’s more like a city streetscape, a complex mesh of roads that merge and intersect with one another at various points.
This video from Dr. Glancy’s lab shows a 3D rendering of the branching contractile structures within a newborn mouse skeletal muscle. Each color represents an individual myofibril segment. The cell membrane (green) and a nucleus (cyan) are also shown.
“It changes the way we understand how the forces that originate in our muscles are transmitted out to the tendons and into the bones,” Dr. Glancy says. “Now that we have found all these little branches, we can follow those forces all the way across the width of the cell, not just the length, and explain how force transmission happens.”
This structure may also account for the ability of muscles to overcome the daily wear and tear of regular use. At the cellular level, our muscles get a little bit damaged all the time. If their myofibrils were completely separate, when one was severed, it would become a dead end for contractile forces, and the negative effects of that break might be quite noticeable.
“Imagine you have a bunch of individual bungee cords in your hands,” Dr. Glancy explains. “If one is cut, it will no longer work because it’s disconnected, but if you have something more like a basketball net, cutting one little piece won’t make a huge difference. You won’t take an entire segment of the muscle out of action. In terms of preventing or minimizing damage, this model better explains the ability of the cells to heal and regenerate. I think this makes more sense.”
Dr. Glancy’s lab is now taking steps to get this new information into biology and medical textbooks. His team is currently working with NIH Medical Arts to create an image that shows the newly understood structure of myofibrils and how it differs from the traditional model. Given the time it usually takes for textbooks to reflect new scientific findings, he hopes to see the new image appear in course materials within the next five years.
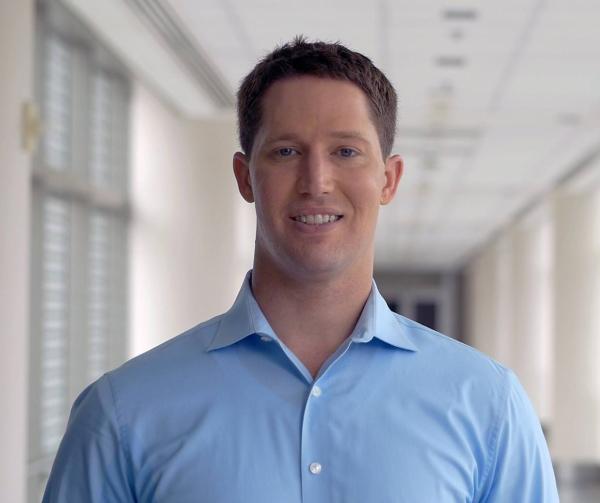
Dr. Brian Glancy
In addition to changing what students learn in the classroom, Dr. Glancy’s discovery could affect the treatments patients receive in the clinic. Having a greater understanding of myofibril structure may provide new directions for understanding and treating diseases that affect our ability to move, such as muscular dystrophy. More common conditions in which muscles aren’t the main players, like diabetes and obesity, are also associated with defects in the production of muscle force, especially among those who are older and less active.
“We already know from our studies that the level of branching is different in various cell types around the body, so that means we may be able to modulate how this connected network functions,” Dr. Glancy says. “We could increase or decrease the amount of branching to make the myofibrils more or less connected in order to improve or repair problems that occur in these diseases.”
To do this, scientists will need to better understand how the extent of branching in myofibrils affects force transmission, as well as the direct implications of changing that transmission.
“Obviously, we’re at a very basic level of understanding right now and any potential treatments are many steps ahead,” Dr. Glancy says, “but this is a new target we can aim at.”
Subscribe to our weekly newsletter to stay up-to-date on the latest breakthroughs in the NIH Intramural Research Program.
References:
[1] The unified myofibrillar matrix for force generation in muscle. Willingham TB, Kim Y, Lindberg E, Bleck CKE, Glancy B. Nat Commun. 2020;11(1):3722. doi: 10.1038/s41467-020-17579-6.
Related Blog Posts
This page was last updated on Tuesday, May 23, 2023