Colleagues: Recently Tenured
LUCY R. FORREST, PH.D., NINDS
Senior Investigator and Section Chief, Computational Structural Biology Section, National Institute of Neurological Disorders and Stroke
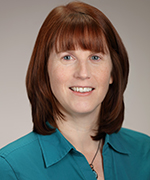
Education: University of Surrey, Guildford, England (B.Sc. in computer-aided chemistry); University of Oxford, Oxford, England (D.Phil. in biochemistry)
Training: Postdoctoral training, Department of Physiology, the Johns Hopkins University School of Medicine (Baltimore); postdoctoral training, Medical Research Council Dunn Human Nutrition Unit (Cambridge, England); postdoctoral training, Center for Computational Biology and Bioinformatics, Columbia University (New York)
Before coming to NIH: Max Planck research group leader, Computational Structural Biology Group, Max Planck Institute for Biophysics (Frankfurt, Germany)
Came to NIH: In 2013 as a tenure-track investigator
Selected professional activities: Reviewing editor, eLife; editorial advisory board, Journal of General Physiology; Faculty of 1000 member in structural biology
Outside interests: Hiking; sailing
Website: https://irp.nih.gov/pi/lucy-forrest
Research interests: Membrane-embedded proteins are essential components of cellular organisms. The proteins allow cells to communicate with their surroundings by providing bridges through the lipid-membrane barrier.
My group is using computational and theoretical approaches to understand the mechanisms of membrane proteins. Of particular interest are transporter proteins, which capture the chemical potential energy of ionic gradients (across the membrane) to facilitate the movement of essential chemicals, or unwelcome toxic compounds, into and out of the cell. A fundamental question is how transporters achieve the required degree of specificity for a given chemical or substrate and how the protein-substrate interaction is coupled to transport ions such as sodium. A further puzzle is how the transporter changes shape to allow access of the substrate binding sites to either side of the membrane, while also preventing leakage.
An essential characteristic of our work is that our hypotheses and interpretations are connected with experimental evidence from biochemical, biophysical, or structural studies. For example, we have long-standing collaborators at Yale University (New Haven, Conn.) with whom we have studied the transport of neurotransmitters such as serotonin, which is crucial to the function of the nervous system. We also collaborate with researchers at several other institutions.
We look for simple rules that underlie very complex biological phenomena. At the same time, to understand these processes at atomic detail, we apply a range of computational tools such as protein-structure prediction and molecular-dynamics simulations. More recently, we have been developing databases to capture the diversity of beautiful, and functionally important, patterns of symmetry that abound in membrane-protein structures.
LAURA BEANE FREEMAN, M.S., PH.D., NCI-DCEG
Senior Investigator, Occupational and Environmental Epidemiology Branch, Division of Cancer Epidemiology and Genetics, National Cancer Institute
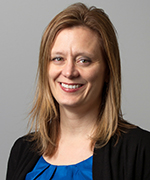
Education: Iowa State University, Ames, Iowa (B.S. in biology); University of Iowa, Iowa City, Iowa (M.S. in preventive medicine; Ph.D. in epidemiology)
Training: Cancer Prevention Fellow, Division of Cancer Prevention and Occupational and Environmental Epidemiology Branch, NCI-DCEG; Research fellow in same branch (2006–2009); became tenure-track investigator in 2009
Came to NIH: In 2003 for training
Selected professional activities: Elected member, EPICOH Management Group; member, Cross-Sector Steering Council for Chronic Diseases and external co-chair for Cancer Agenda, National Occupational Research Agenda (NORA), National Institute for Occupational Safety and Health; Cancer Sub-Group chair, AGRICOH (an international consortium of agricultural cohort studies)
Outside interests: Reading; traveling; cooking
Website: https://irp.nih.gov/pi/laura-beane-freeman
Research interests: I conduct research on several occupational and environmental exposures including pesticides and other agricultural agents; drinking-water contaminants, such as disinfection byproducts; and formaldehyde and other industrial chemicals.
In the agricultural realm, studies around the world have found that farmers and other agricultural workers are at elevated risk of several specific cancers including Hodgkin lymphoma, non-Hodgkin lymphoma, leukemia, multiple myeloma, and cancers of the brain, skin, lip, stomach, and prostate. Work-related exposures suspected of contributing to the excesses include pesticides, viruses, mycotoxins, and well-water contaminants.
I am the NCI principal investigator of the Agricultural Health Study (AHS)—a collaboration of the NCI, the National Institute of Environmental Health Sciences, the National Institute for Occupational Safety and Health, and the Environmental Protection Agency. The AHS is a prospective cohort study of approximately 90,000 participants. The participants include licensed private pesticide applicators and their spouses in Iowa and North Carolina, and commercial applicators in Iowa. We are investigating how agricultural exposures including pesticides, but also exposure to livestock and poultry, diesel exhaust, endotoxins and other bioaerosols, influence cancer risk. There is increasing evidence that early-life exposures may influence disease risk in adulthood. Therefore, I have initiated a study of offspring of the AHS participants to investigate how their childhood farm exposures may affect their health in later life.
In the drinking-water contaminants realm, previous studies have shown that exposure to disinfection byproducts (DBPs) increases the risk of bladder cancer. However, DBPs are a complex mixture of chemicals, so questions remain about the etiologic agents responsible for the associations. Recent results from a case-control study suggest that in addition to ingestion, exposure through showering and bathing may contribute to risk. In a large case-control study in the United States, we investigated exposure from multiple routes and found evidence that brominated DBPs may be of particular concern.
My research with formaldehyde has involved assessing whether occupational exposure to formaldehyde increases the risk of cancer at several sites, specifically the respiratory tract, and whether it induces lymphohematopoietic malignancies. We are also evaluating the biologic plausibility of formaldehyde carcinogenicity, particularly at sites distant from the respiratory tract. The NCI Cohort of Workers in Formaldehyde Industries is the largest study of occupationally exposed workers and includes more than 25,000 participants who have a median follow-up time of over 40 years. Our most recent follow-up on this study offered further insight into the temporality of potential associations between formaldehyde and leukemia and other lymphohematopoietic malignancies.
MICHAIL S. LIONAKIS, M.D., SC.D., NIAID
Senior Investigator and Chief, Fungal Pathogenesis Section, Laboratory of Clinical Microbiology and Immunology, National Institute of Allergy and Infectious Diseases
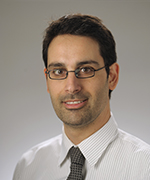
Education: University of Crete Medical School, Heraklion, Greece (M.D.); University of Crete, Heraklion (Sc.D.)
Training: Rural service physician, Greek Ministry of Health and Welfare (Katerini, Greece); postdoctoral research fellow, Department of Infectious Diseases, Infection Control and Employee Health, University of Texas M. D. Anderson Cancer Center (Houston); internal medicine resident, Department of Internal Medicine, Baylor College of Medicine (Houston); infectious diseases clinical fellow, NIAID
Came to NIH: In 2007 for training; became assistant clinical investigator in 2010, clinical tenure-track investigator in 2012
Selected professional activities: Member of several editorial boards including JCI Insight and Journal of Infectious Diseases; associate editor, Frontiers in Cellular and Infection Microbiology; faculty member, Faculty of 1000–Immunology, Immunity to Infections
Outside interests: swimming; music; dancing; traveling
Website: https://irp.nih.gov/pi/michail-lionakis
Research interests: Since the 1990s, fungal infections have emerged as a major cause of morbidity and mortality in immunosuppressed and critically ill patients. The yeast genus Candida is the most common human fungal pathogen, and the mold genus Aspergillus is the leading cause of infectious mortality in transplant recipients.
My laboratory research focuses on 1) the cellular and molecular factors that regulate the immune response against mucosal and invasive candidiasis and invasive aspergillosis in clinically relevant animal models; 2) a better understanding of the genetic and immune defects that underlie enhanced susceptibility to mucocutaneous and invasive fungal infections in humans.
Our goal is to develop a detailed mechanistic understanding of the molecular and cellular basis of innate and adaptive immune responses against Candida and Aspergillus. We aim to devise novel strategies to improve the diagnosis of fungal infections and augment or supplement the current antifungal drug treatment. To study host-fungal interactions, we are using in vitro cell-culture systems and clinically relevant mouse models of mucosal and systemic Candida and Aspergillus infections. Our research techniques include a variety of immunological, biological, and imaging approaches.
The molecular factors that mediate the immune response to candidiasis and aspergillosis are poorly defined. So my lab is investigating the molecular cues that mediate trafficking and effector function of specific resident and recruited immune cells in antifungal host defense in vivo. We are also interested in delineating the host factors that govern fungus-specific resistance versus susceptibility at different anatomical sites.
In our clinical-research program, we are investigating the mechanisms of fungal susceptibility in inherited immunodeficiencies that lead to invasive pulmonary and brain fungal infections or to chronic mucocutaneous candidiasis. We have enrolled the world’s largest cohort of patients with genetic immune disorders—deficiency of caspase recruitment-domain-containing protein 9 and deficiency of autoimmune regulator—that make them susceptible to fungal infections. By using corresponding gene-deficient mice and patients with susceptibility to severe or recurrent fungal disease, we hope to discover novel genetic variants associated with this susceptibility and to develop mechanism-based therapies for people affected by fungal infections.
ANTONINA ROLL-MECAK, PH.D., NINDS
Senior Investigator and Chief, Cell Biology and Biophysics Unit, National Institute of Neurological Disorders and Stroke
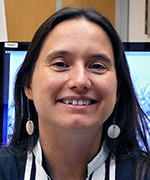
Education: Albert E. Nerken School of Engineering, The Cooper Union for the Advancement of Science and Art, New York (B.E. in chemical engineering); The Rockefeller University, New York (Ph.D. in molecular biophysics)
Training: Postdoctoral fellow, Department of Cellular and Molecular Pharmacology, University of California, San Francisco (San Francisco)
Came to NIH: In 2010
Selected professional activities: Referee for several journals including Journal of Cell Biology, Cell, Nature, and Science; faculty member, Faculty of 1000 Cell Signaling and Trafficking Structures Section
Outside interests: Loves classical music so she tries to squeeze in a concert whenever she can; also enjoys the great museums in Washington, D.C.
Website: https://irp.nih.gov/pi/antonina-roll-mecak
Research interests: I am interested in the morphology and dynamics of the microtubule cytoskeleton. Microtubules provide structural support to cells as well as form a complex and dynamic intracellular “highway” that delivers molecular cargo from one end of the cell to the other.
In particular, I am trying to understand how the chemical and genetic diversity of tubulin—the protein building blocks of microtubules—modulates the microtubule network. Microtubules are involved in biological processes throughout the body such as cell division, motility, and differentiation; microtubule disturbances underlie many neurodegenerative disorders such as Alzheimer and Parkinson diseases as well as cancer.
Deceptively uniform ultrastructurally, microtubules are composed of multiple tubulin isoforms that bear a bewildering range of post-translational modifications including acetylation, detyrosination, phosphorylation, glutamylation, and glycylation. Tubulin alpha and beta heterodimers consist of a compact folded body and intrinsically disordered COOH-terminal tails. These tails form a dense lawn on the microtubule surface and serve as binding sites for molecular motors and microtubule-associated proteins. The majority of sequence variation and post-translational modifications of tubulin isoforms concentrates on these intrinsically disordered tails. Some of the modifications we study, such as glutamylation and glycylation, can add amino-acid chains that are significantly longer than the tubulin tails themselves. These modifications are thought to constitute a “tubulin code” (analogous to the histone code), which is read by cellular effectors.
To crack the code, we first need to understand how it is written: the mechanism and regulation of the enzymes that introduce tubulin-post-translational modifications.
Second, we need to elucidate how post-translational modifications and isoform variability affect the basic properties of the microtubule polymer itself. Third, we need to know how the spatial and temporal patterns of microtubule modifications are established and propagated.
Ultimately, we need to understand how tubulin isoform composition and modifications are interpreted by the cellular effectors that regulate their recruitment or activity.
To provide a mechanistic look at the tubulin code, my laboratory has developed a biochemical platform to obtain recombinant, isotypically pure human tubulin as well as quantitatively defined, differentially modified tubulins. We use a variety of techniques to decipher the code, from structural biology and classic enzymology to single-molecule biophysics, live-cell imaging, and modeling.
JUSTIN W. TARASKA, PH.D., NHLBI
Senior Investigator, Laboratory of Cellular Biophysics, Biochemistry and Biophysics Center, National Heart, Lung, and Blood Institute
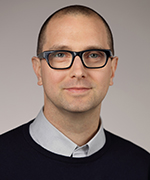
Education: Reed College, Portland, Ore. (B.A. in biology); Vollum Institute, Oregon Health and Science University, Portland (Ph.D. in cell biology)
Training: Postdoctoral training, Department of Physiology and Biophysics, University of Washington (Seattle)
Came to NIH: In 2010
Selected professional activities: Editorial board, Journal of General Physiology; director, Analytical and Quantitative Light Microscopy course, Marine Biological Laboratory (Woods Hole, Mass.); recipient of Presidential Early Career Award for Scientists and Engineers (2012)
Outside interests: Spending time with family; drawing; aikido; running; gardening; surfing
Website: https://irp.nih.gov/pi/justin-taraska
Research interests: My lab develops and uses advanced fluorescence and electron microscopy imaging methods to investigate the molecular organization of proteins that regulate exocytosis and endocytosis in human cells.
Cells communicate with each other and the body by releasing proteins, peptides, and chemicals through a highly regulated process called exocytosis, in which cytoplasmic cargo-loaded vesicles fuse with the cell’s plasma membrane and release their contents. Cells go to great lengths to ensure that exocytosis occurs at precisely the right time and location and that the correct quantity and type of materials are released. Once vesicles fuse, vesicle material is cleared from the plasma membrane through a coat-driven process called endocytosis, in which the plasma membrane folds inward to bring substances into the cell. The primary retrieval mechanism in eukaryotic cells is clathrin-mediated endocytosis (CME). In CME, dozens of proteins capture, polymerize, and bend a honeycomb-like coat around pieces of the plasma membrane to internalize material. But scientists don’t yet understand how this complex ensemble of proteins is organized.
In a healthy cell, the exocytosis and endocytosis processes are carefully balanced. Disruption of either can result in deficiencies or excesses of chemical signals, leading to such disorders as Parkinson and Huntington diseases, schizophrenia, epilepsy, diabetes, and heart and lung diseases. Not surprisingly, understanding exocytosis and endocytosis has been a major goal for the biological sciences. A gap exists between understanding protein structures and their cellular contexts. We aim to fill this gap by developing and using imaging tools to determine the nanoscale structures, organization, and dynamics of molecules that are important for the biology of membrane traffic. My lab maps the fundamental architecture of molecular machines to understand how these complex assemblies function.
This page was last updated on Friday, April 8, 2022