How a Marker for Genetic Damage Changed the Study of DNA
Decades Later, IRP Researcher’s Discovery Is Used in Labs Around the World
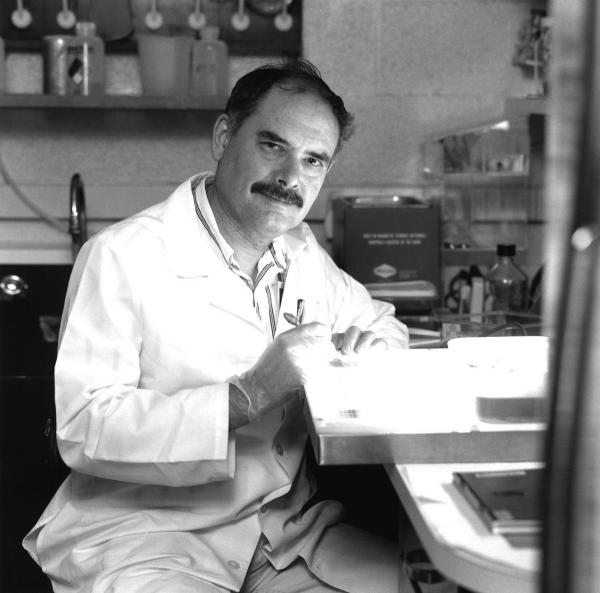
Photo by Bill Branson.
Dr. William Bonner’s research at the NIH revolutionized the study of DNA repair by allowing scientists to detect a form of DNA damage called a double-strand break.
National DNA Day, held on April 25, commemorates the completion of the Human Genome Project in 2003 and the day in 1953 when a research team led by Drs. James Watson, Francis Crick, Maurice Wilkins, and Rosalind Franklin published their groundbreaking paper on the structure of DNA in the journal Nature.
The mapping of DNA’s structure opened the door to modern genetics and our current understanding of how DNA affects the health and survival of all living things. Since then, there have been numerous additional major leaps forward in the field of genetics. Among them was the discovery of a universal hallmark of DNA damage by IRP Scientist Emeritus William Bonner, Ph.D., an advance that revolutionized the study of how cells sense and repair genetic defects. Dr. Bonner’s findings paved the way for a deeper understanding of cell biology, as well as clinical advances for treating cancer and for assessing risks from radiation in the environment.
When Dr. Bonner came to the NIH in 1974, he brought with him a keen interest in histones, the proteins that DNA coils around so it can fit inside the cell nucleus. In 1980, while working at the NIH’s National Cancer Institute (NCI), he discovered two previously unnoticed variants to one of the four ‘core’ histones, known as H2A. He named these variants H2AX and H2AZ and set about trying to determine their function.
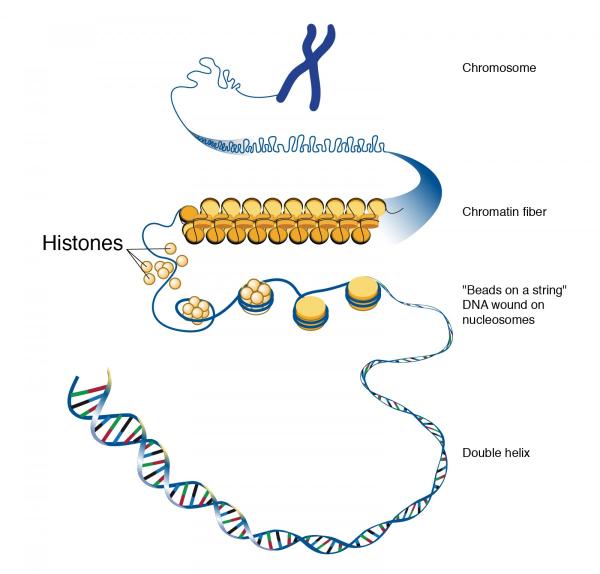
Segments of DNA wind around histones to form bundles called nucleosomes, which are squished together in a substance called chromatin in order to fit all of a cell’s DNA into its nucleus.
“My research group was one of only a handful looking at histones,” Dr. Bonner says. In fact, he remembers that histones once won a biotech company publication’s contest for the world’s most boring proteins.
Yet the new histone variants Dr. Bonner identified proved to be anything but boring. He found closely related versions of them in everything from yeast to human cells, which led him to wonder if they played an important role that had been preserved throughout evolution. Thinking that H2AX may have something to do with basic cell survival, he used various means to stress cells in order to see what would happen to H2AX. He didn’t have much luck until he exposed the cells to ionizing radiation, which induces a particularly harmful form of DNA damage called a double-strand break. When such a break occurs, it’s like losing part of the cell’s instruction manual. Regular activity breaks down, leaving the cell susceptible to the types of malfunctions that can kill cells or lead to cancer.
“It was serendipitous that we had a radiation machine in the basement,” Dr. Bonner remembers. “I could see a huge change — about fifty percent of the H2AX histones had been converted into something else.”
Dr. Bonner’s team found that the new form of H2AX induced by radiation came about due to a modification called phosphorylation. Phosphorylation happens when a chemical tag called a phosphoryl group, an electrically charged collection of oxygen and phosphorus atoms, attaches to a molecule. This often changes the molecule’s function. In the case of an H2AX histone, the presence of a double-strand break in the DNA coiled around the histone triggers a chemical reaction that causes a phosphoryl group to attach to the histone. Dr. Bonner’s team named this phosphorylated form of the histone ‘gamma-H2AX.’
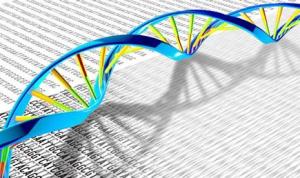
More From the IRP
Blog
Plugging the Gaps in the Human Genome
Until Dr. Bonner’s discovery, which was published in 1998, it was difficult to study damaged sections of the genome. To see a double-strand break, researchers had to bombard cells with lethal doses of radiation that caused massive damage. Of course, if the cell can’t survive, it is very difficult to study cellular changes that result from non-lethal damage.
By tagging gamma-H2AX histones with a fluorescent antibody, Dr. Bonner showed that exposing cells to ionizing radiation caused hundreds of H2AX histones near the site of a double-strand break to become phosphorylated within minutes, creating a rapid and highly amplified response akin to a cellular fire alarm. At long last, scientists could finally observe non-lethal accumulations of double-strand breaks and the steps cells take to repair them under the microscope.
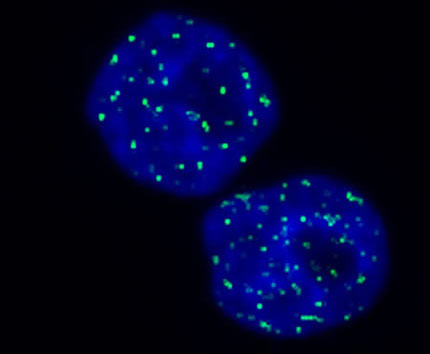
Dr. Bonner’s technique for tagging gamma-H2AX histones with a fluorescent molecule allows researchers to identify locations (green) in cells where DNA double-strand breaks are being repaired.
Dr. Bonner and his research team subsequently began collaborating with an NCI colleague, Andre Nussenzweig, Ph.D., to develop ‘knockout’ cells and mice that lacked H2AX histones. While these cells and animals survived, they proved to be more prone to developing mutations in their DNA after exposure to radiation, revealing that H2AX is essential for the proper repair of DNA double-strand breaks. What’s more, even when they were not exposed to radiation, the H2AX knockout mice exhibited two other problems that showed the importance of double-strand breaks that occur naturally in the body. First, the mice had generally poor health because their cells could not complete the normal DNA rearrangements necessary for a robust immune system. Second, male mice were sterile because they could not produce sperm. In this way, double-strand breaks are a double-edged sword: random, unwanted breaks can result in damaging mutations when they are repaired, but breaks formed deliberately and temporarily during certain processes are necessary for good health.
This multifaceted nature of DNA double-strand breaks makes them a topic of wide and intense scientific interest. Today, the technique that Dr. Bonner developed to detect gamma-H2AX is used to study double-strand breaks in laboratories all over the world: in basic science research, studies of disease, therapeutic development, and measurements of risk due to radiation exposure.
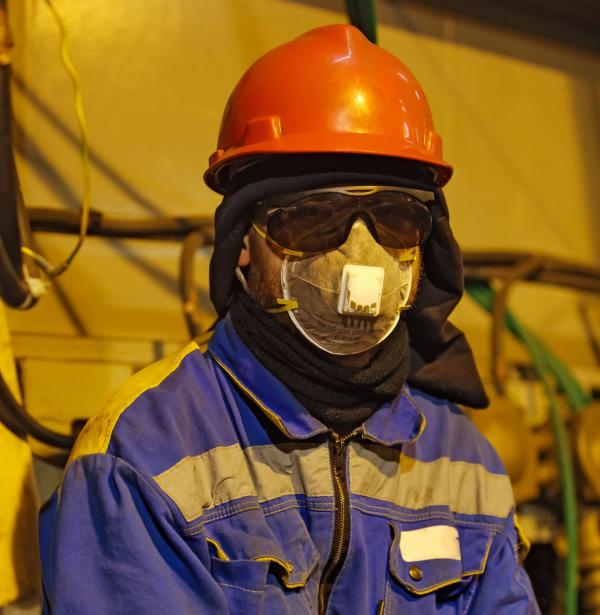
Dr. Bonner’s method for detecting gamma-H2AX histones has found applications in a wide range of fields, including studies of the risks faced by workers exposed to radiation.
“Many people would call me up and arrange a collaboration to see if gamma-H2AX was involved in something they were studying,” Dr. Bonner says.
For example, a researcher in England wanted to collaborate on an experiment to see if gamma-H2AX is involved in cell division. (It is.) Meanwhile, other groups are using Dr. Bonner’s technique to test whether experimental drugs damage DNA. It can also be used to assess damage to DNA in cancer patients’ blood samples to determine how well their treatment is working, since most cancer treatments work by destroying the DNA of cancer cells. The U.S. Army is even using it to study the effects of accidents that expose people to radiation.
“My goal early on in my career was to make a seminal discovery of some sort,” reflects Dr. Bonner, who is now retired but maintains an ongoing working relationship with the NIH as a Scientist Emeritus. “I did that, so yeah, I guess I reached my goal.”
Subscribe to our weekly newsletter to stay up-to-date on the latest breakthroughs in the NIH Intramural Research Program.
References
[1] GammaH2AX and cancer. Bonner WM, Redon CE, Dickey JS, Nakamura AJ, Sedelnikova OA, Solier S, Pommier Y. Nat Rev Cancer. 2008; 8(12):957-67.
[2] A critical role for histone H2AX in recruitment of repair factors to nuclear foci after DNA damage. Paull TT, Rogakou EP, Yamazaki V, Kirchgessner CU, Gellert M, Bonner WM. Curr Biol. 2000; 10(15):886-95.
[3] Megabase chromatin domains involved in DNA double-strand breaks in vivo. Rogakou EP, Boon C, Redon C, Bonner WM. J Cell Biol. 1999; 146(5):905-16.
[4] DNA double-stranded breaks induce histone H2AX phosphorylation on serine 139. Rogakou EP, Pilch DR, Orr AH, Ivanova VS, Bonner WM. J Biol Chem. 1998; 273(10):5858-68.
[5] Two-dimensional gel analysis of histones in acid extracts of nuclei, cells, and tissues. Bonner WM, West MH, Stedman JD. Eur J Biochem. 1980; 109(1):17-23.
[6] Histone 2A, a heteromorphous family of eight protein species. West MH, Bonner WM. Biochemistry. 1980; 19(14):3238-45.
Related Blog Posts
This page was last updated on Tuesday, May 23, 2023