Repurposed Drugs Could Curb Cancer Drug Resistance
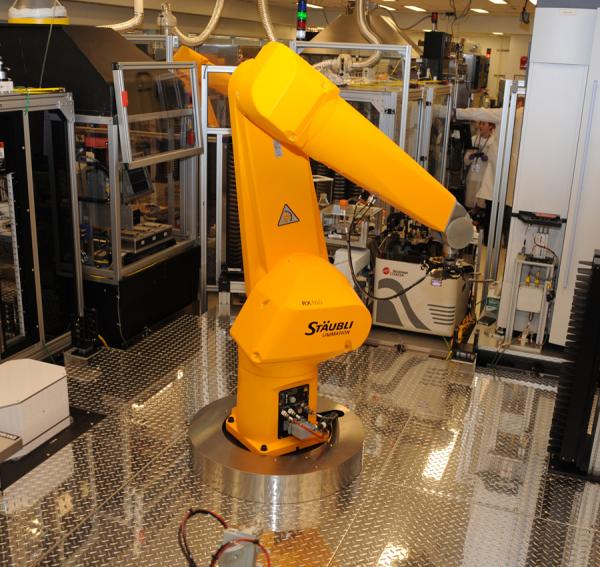
Robots like this one are a critical part of the high-throughput drug screening technique that IRP researchers recently used to uncover one way ovarian cancer can become resistant to chemotherapy.
Much of the time, new therapies are built from the ground up, with researchers closely scrutinizing a specific molecule or cellular process and designing compounds that can influence it. In some cases, however, scientists take the opposite approach, throwing a multitude of therapeutic darts at the disease dartboard to see what sticks, and then working backwards to unravel why a drug was effective. IRP researchers recently used this method to identify potential treatments for drug-resistant ovarian cancer and determine how some of those tumors become impervious to a particular chemotherapy.1
The first-line treatment for ovarian cancer is the chemotherapy drug cisplatin. While the treatment is often highly effective at first, in a majority of patients the cancer returns within a few years and is no longer vulnerable to cisplatin. This is a key reason why death rates for ovarian cancer have declined much more slowly over the past several decades than mortality rates for many other cancers.
In the new study, a team of IRP scientists led by Wei Zheng, Ph.D., used a technique called a high-throughput drug repurposing screen to test the effect of more than six thousand pharmacologic compounds on a particular strain of cisplatin-resistant ovarian cancer cells developed by Wenge Zhu, Ph.D., a former IRP postdoctoral fellow who now runs a lab at the George Washington University School of Medicine. The compounds tested in the screen included roughly 1,500 FDA-approved drugs; several hundred therapeutics approved for use in other countries like Canada and Japan; a number of drugs currently being tested in FDA clinical trials for cancer and other diseases; and a wide array of compounds used for treating illness in animals or in topical products like shampoos.
“It takes an average of 12 years and hundreds of millions of dollars to develop a new drug from scratch,” Dr. Zheng says. “A drug repurposing screen is a shortcut. You use drugs that have already been approved for use in humans or animals for new disease indications, avoiding the long, expensive drug development process.”
The researchers first tested whether each compound could kill the cisplatin-resistant cancer cells when combined with cisplatin, ultimately identifying 383 that were able to do so. However, that experiment could not determine whether those compounds were effective on their own or whether they worked by restoring the cells’ vulnerability to cisplatin, so the research team tested those 383 compounds again by themselves and in combination with cisplatin. This second step found 38 pharmacologic agents that inhibited the cancer cells’ growth by themselves when used at clinically relevant doses. It also identified 12 compounds that were only effective against the cancer when combined with cisplatin, suggesting they worked by re-sensitizing the cancer to cisplatin. Five of these are known to be capable of reaching clinically useful concentrations in human blood, making them promising partners for cisplatin treatment in patients with chemotherapy-resistant ovarian cancer.
Because one of those five drugs, called CUDC-101, is known to inhibit a cellular protein called the epidermal growth factor receptor (EGFR), the researchers wondered whether blocking that protein played a role in re-sensitizing the cancer cells to cisplatin. Further experiments revealed that, compared to cancer cells that remained vulnerable to cisplatin, the cisplatin-resistant cells had higher levels of both EGFR and its active form, phosphorylated EGFR. In addition, cisplatin treatment by itself dramatically increased levels of phosphorylated EGFR in the cancer cells, while CUDC-101 treatment markedly reduced them. Finally, suppressing the activity of the gene that produces EGFR, as well as exposing the cancer cells to three other known EGFR inhibitors, restored cisplatin’s ability to kill the resistant cancer cells.
“That’s further proof that EGFR in that particular cell line is an important part of cisplatin resistance,” says Dr. Zheng. “This molecule is a growth receptor — it promotes cell growth, so you can imagine it also promotes cancer cell growth. That makes it a clear drug target.”
EGFR likely contributes to treatment resistance in only a subset of tumors, which could explain why EGFR inhibitors have failed in past clinical trials for drug-resistant cancers. Indeed, other sets of cisplatin-resistant ovarian cancer cells have been found to develop their resistance through entirely different mechanisms.2,3 Consequently, for the combination of EGFR inhibitors and cisplatin to be a useful treatment, doctors will have to first confirm that a patient’s cisplatin-resistant cancer also shows increased EGFR levels.
“Our findings provide further confirmation of the variation in resistant cancer,” says Dr. Zheng. “That could be significant in the future for precision medicine. This study indicates the importance of individualized treatment, especially for patients with drug-resistant cancer.”
Subscribe to our weekly newsletter to stay up-to-date on the latest breakthroughs in the NIH Intramural Research Program.
References:
[1] Small molecules identified from a quantitative drug combinational screen resensitize cisplatin’s response in drug-resistant ovarian cancer. Sima N, Sun W, Gorshkov K, Shen M, Huang W, Zhu W, Xie X, Zheng W, Cheng X. Transl Oncol. 2018 Jul 4;11(4):1053-1064. doi: 10.1016/j.tranon.2018.06.002.
[2] DUOXA1-mediated ROS production promotes cisplatin resistance by activating ATR-Chk1 pathway in ovarian cancer. Meng Y, Chen CW, Yung MMH, Sun W, Sun J, Li Z, Li J, Li Z, Zhou W, Liu SS, Cheung ANY, Ngan HYS, Braisted JC, Kai Y, Peng W, Tzatsos A, Li Y, Dai Z, Zheng W, Chan DW, Zhu W. Cancer Lett. 2018 Aug 1;428:104-116. doi: 10.1016/j.canlet.2018.04.029.
[3] Autocrine activation of JAK2 by IL-11 promotes platinum drug resistance. Zhou W, Sun W, Yung MM, Dai S, Cai Y, Chen CW, Meng Y, Lee JB, Braisted JC, Xu Y, Southall NT, Shinn P, Huang X, Song Z, Chen X, Kai Y, Cai X, Li Z, Hao Q, Cheung ANY, Ngan HYS, Liu SS, Barak S, Hao J, Dai Z, Tzatsos A, Peng W, Pei H, Han Z, Chan DW, Zheng W, Zhu W. Oncogene. 2018 Jul;37(29):3981-3997. doi: 10.1038/s41388-018-0238-8.
Related Blog Posts
This page was last updated on Tuesday, January 30, 2024