Let There Be Light!
Optogenetics, a new technology used to control brain activity with light, has revolutionized the field of neuroscience in the past decade. The combination of two powerful tools, genetics and optics, has provided both temporal and spatial acuity in understanding how the brain works in response to sensory and motor cues in the environment. At the recent NIH Research Festival symposium titled “Optogenetic approaches to investigating the nervous system,” fellows and scientists from the NIH community presented their research encompassing topics that make use of this approach to study different systems.
The opening presentation was by Kimberly LeBlanc, a postdoctoral fellow at NIDDK and one of this year’s FARE award winners, who shared her work on deciphering the brain circuitry underlying anxiety-like behaviors. She studies the role of indirect pathway medium spiny neurons (iMSNs), which project from the striatum, a component of the basal ganglia. This falls under the domain of the dopaminergic circuitry that is thought to be involved in stress and depression. Using both in vivo electrophysiology and c-fos immunohistochemistry, she is trying to detect the striatum’s role in anxiety. On stimulating the iMSNs, movement in animals was greatly reduced in an elevated zero maze, a model used to test anxiety in rodents, along with an increase in anxiety-like behaviors. Conversely, stimulation of the direct pathway medium spiny neurons (dMSNs) showed opposite effects on speed and anxiety.
By using the same technique, Hadley Bergstrom, a postdoctoral fellow at NIAAA and a FARE award winner, explores how reward learning is controlled by the dorsal striatum. Dubbed the network “hub” for generating reward memories, the dorsal striatum receives input from different cortical structures and is further subdivided into dorsal medial striatum (DMS) and dorsal lateral striatum (DLS). While DMS is essential for goal-directed behavior, DLS is necessary for habitual actions. This was further corroborated by the present study, which showed that DMS supports learning and reward acquisition, and DLS interferes with these processes. In fact, silencing the DLS during a specific phase of a behavioral task in rodents facilitates learning. In conclusion, these functional differences could possibly arise due to diversity in afferent connectivity of DLS and DMS.
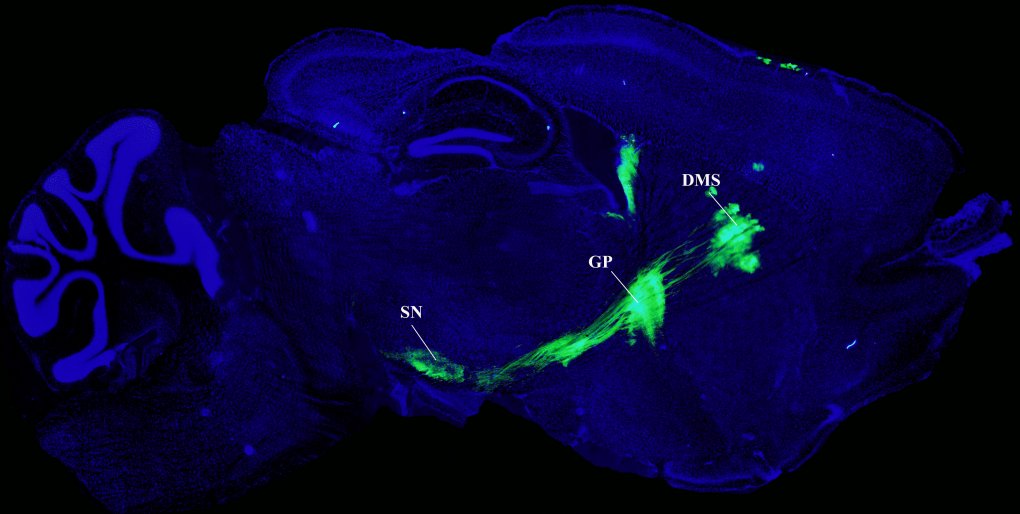
rAAV8/CAG-ArchT-GFP (a viral construct) injected into the dorsal medial striatum (DMS) illuminates the direct/indirect pathways of the substantia nigra (SN) and globus pallidus (GP). Image courtesy of Hadley Bergstrom.
Ross McDevitt, a postdoctoral fellow at NIDA, also explores the reward circuitry and explained the role of heterogeneous cell populations in the dorsal raphe nucleus (DRN) that modulate reward-motivated behaviors. The DRN is a structure in the brainstem that mainly comprises serotonin-producing neurons and is involved in learning behaviors. However, it is the nonserotonergic neurons in DRN that, upon optogenetic stimulation, promote reward learning. These neurons primarily project to the ventral tegmental area in the midbrain and reinforce reward-based learning. Additionally, this study disproves the idea that DRN serotonin is rewarding.
From behaviors in rodents, the presentation moved onto understanding movements using neonatal mice. Melanie Falgairolle, a research fellow at NINDS, uses optogenetics to study how the central pattern generators work and the involvement of motoneurons in locomotion. Central pattern generators are neural networks that underlie the production of rhythmic motor patterns, such as walking, breathing, and swimming. This study will shed some light on how the spinal cord circuitry modulates rhythmic movements and what neurons are responsible for this process.
Another tool used to interrogate brain functions is chemogenetics, a technique used by Zhenzhong Cui, a researcher at NIDDK. Chemogenetics is being used to explore the role of agouti-related protein (AgRP) neurons in feeding behavior in mice. These neurons are located in the hypothalamus and are thought to be involved in energy homeostasis. The intracellular signaling mechanism that leads to an increase in chronic food intake and weight gain involves activation of these AgRP neurons via Gs-coupled receptor activation. Drugs that inhibit this cascade could ultimately help in controlling obesity by inactivating the AgRP neurons.
Thanks to the wide variety of presenters, this Research Festival symposium showcased the diverse work being conducted by talented NIH scientists. Each of the researchers uses cutting-edge tools to unlock the secrets of how the central nervous system controls our behaviors, with effects ranging from anxiety and learning to feeding and movement.
To learn more about optogenetics and engage in discussions on advances and applications of the technology, join the Optogenetics Interest Group at NIH.
Related Blog Posts
This page was last updated on Wednesday, January 31, 2024