Research Briefs
Read about NIH scientific advances and discoveries by intramural scientists: experimental cancer vaccine slows tumor growth; and live tracking of immune cell signaling; how cadmium exposure affects heart development; bridging the gap between genetics and ADHD; how brain activity is fine-tuned; some cancer and autoimmunity drugs may increase susceptibility to sars-cov-2 infection.
NIAID: EXPERIMENTAL CANCER VACCINE SLOWS TUMOR GROWTH IN MICE
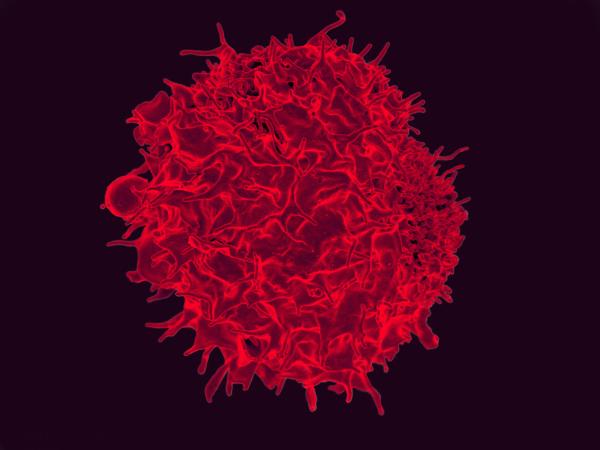
CREDIT: NIAID
NIAID: A recent study by NIAID scientists and their collaborators has yielded a promising cancer vaccine that reduced colorectal tumor growth in mice. Shown: colorized scanning electron micrograph of a T lymphocyte.
A recent study by NIAID scientists at the Vaccine Research Center and their collaborators at Vaccitech North America (Baltimore) has yielded a promising cancer vaccine that reduced colorectal tumor growth in mice. The treatment works much like a normal vaccine by priming the immune system to target an antigen—in this case a tumor-derived protein. While most vaccines promote antibody production, this vaccine, named SNAPvax, instead prepares cytotoxic killer T cells to specifically recognize and attack tumors. A critical requirement of this vaccine to mediate protection was to administer it intravenously rather subcutaneously.
The investigators also discovered that the intravenous delivery of the vaccine circumvents the tumor immunosuppression common in local tumor environments. This was due to the vaccine’s adjuvant (an additive used to stimulate the immune system to react to the antigen) by increasing the production of type I interferons (IFN-I) in the blood and tumor, which are important immune-system-signaling molecules. The production of IFN-I reversed tumor-induced immunosuppression. By effectively removing the brakes induced by the body’s immune response to the tumor, the killer T cells faced fewer obstacles to mounting their attack. Vaccitech North America plans to develop the platform as a treatment for human papillomavirus cancers in 2023. (NIH authors: F. Baharom, R.A. Ramirez-Valdez, M. Dillon, D. Hermans, S. Fussell, K.K.S. Tobin, A.S. Ishizuka, and R.A. Seder, Cell 185:4317-4332.e15, 2022)
NIA, NIAID, NCI: LIVE TRACKING OF IMMUNE CELL SIGNALING
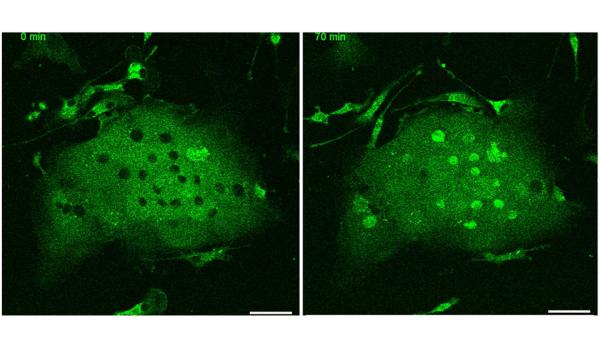
CREDIT: MYONG-HEE “MIA” SUNG, NIA
NIA: NF-κB RelA protein, visualized as green fluorescent protein, was monitored in live immune cells, or microglia, from the brain of a knock-in mouse. The left image was taken before stimulation with the inflammatory tumor necrosis factor.
For the first time, scientists were able to study real-time behaviors of nuclear factor-kappa B (NF-kappa-B), a protein involved in regulating immune and inflammatory responses, in active inflammatory cells. An NIA-led team of researchers developed mouse strains that allow researchers to monitor this important protein’s activity.
“Since NF-kappa-B influences virtually all physiological processes and inflammatory diseases, understanding exactly how this protein works is essential for precision medicine,” said corresponding author Myong-Hee “Mia” Sun (NIA).
The NF-kappa-B family comprises five structurally related proteins, and this study examined two major forms, RelA and c-Rel. Using molecular biology techniques to attach a green or red fluorescent marker to RelA and c-Rel, the scientists knocked-in each of these constructs to create four strains of mice. By crossbreeding the mice, the researchers also created a double knock-in strain in which both proteins are fluorescently labeled.
The team found differences in how cells communicated with each other depending on whether the cells were primary cells, taken directly from tissue, or immortalized cells, grown in a lab. The data also showed as the mice aged, c-Rel had a more prominent role for inflammatory responses in microglia, which are brain immune cells implicated in neuroinflammatory diseases, such as Alzheimer’s. In addition, isolates of various tissues established that RelA and c-Rel amounts decrease at certain stages of maturity for immune cells, such as T lymphocytes and myeloid cells. Sung hoped these mice would be a great resource for advancing research in aging, inflammatory diseases, and immunity. (NIH authors: S.M.T. Rahman, M. Aqdas, E.W. Martin, F. Tomassoni-Ardori, P. Songkiatisak, K.-S. Oh, S. Uderhardt, Q.C. Claybourne, R.A. McDevitt, R.N. Germain, L. Tessarollo, and M.H. Sung, Cell Rep 41:111682, 2022)
NIEHS: NEW TOOLS REVEAL HOW CADMIUM EXPOSURE AFFECTS HEART DEVELOPMENT
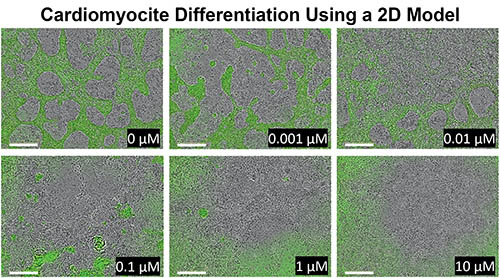
CREDIT: NIEHS
NIEHS: 2D model showing how the pluripotent stem cells react to human relevant doses of cadmium over 8 days. From the control in the first panel, to the last panel, researchers can see how the differentiation to cardiomyocytes is inhibited with different doses of cadmium.
Researchers at NIEHS have developed three different cell models to better understand the link between low doses of the metal cadmium (Cd) and cardiovascular disease. Cd is a common environmental exposure, derived from refining and manufacturing sources. It has been associated with conditions such as congenital heart disease, the most common type of birth defect.
The investigators first studied how Cd exposure can affect formation of the mesoderm—one of the three germ layers in animal embryos—by using a three-dimensional model made of embryonic stem cells (ESCs), which can turn into any type of cell in the body. A two-dimensional cell model was then used to determine how Cd affects cardiac differentiation: Levels of NKX2, a protein known to be involved in heart development, were measured. The scientists used an ESC line containing a green fluorescent protein attached to the NKX2 gene to measure differentiation to cardiomyocytes. Finally, they used another 3D model to simulate the beating heart and investigate how Cd affects normal heart movement and function.
From the three models, the research team found that exposure to low doses of Cd can reduce cardiomyocyte differentiation by suppressing a critical pathway involved in cell-fate determination, reduce amounts of NKX2 and other cardiac-specific genes, and inhibit proper heart function. This study gives insight into the mechanisms behind Cd-induced heart abnormalities and provides a new tool for studying other environmental exposures and their disease associations. (NIH authors: X. Wu, Y. Chen, A. Luz, G. Hu, and E.J. Tokar, Environ Health Perspect 130:117002-1 to 117002-11, 2022)
NHGRI, NIMH: BRIDGING THE GAP BETWEEN GENETICS AND ADHD
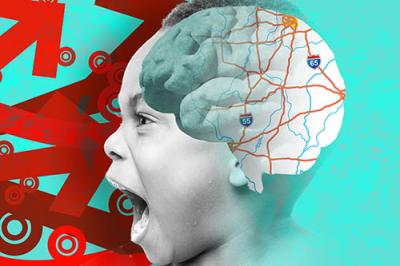
CREDIT: DARRYL LEJA, NHGRI
NHGRI: ADHD and Brain Mapping. White matter contains the majority of nerve fibers in and transfers information across the brain. With the help of magnetic resonance imaging (MRI) and advanced image processing, researchers can map connections in the brain and determine whether there are subtle disruptions to the white matter tracts in people with Attention-Deficit Hyperactivity Disorder (ADHD).
By demonstrating differential gene expression in regions of the brain associated with attention-deficit hyperactivity disorder (ADHD), scientists at NHGRI and NIMH have made a significant step toward connecting the dots between the disorder’s symptoms and genetics.
ADHD, one of the most common neurodevelopmental disorders of childhood, affects over 3% of adults and 7% of children globally. The symptoms of ADHD commonly include inattention, hyperactivity, or impulsivity and can interfere with various aspects of life. While there are known genetic risk factors as well as brain regions and activity that are associated with the disorder, a direct connection between them has been lacking.
In this study, researchers compared RNA-sequencing data from the postmortem brain tissue of individuals with or without an ADHD diagnosis. They examined gene expression in two brain regions implicated in ADHD: the caudate and the anterior cingulate cortex (ACC). In the ACC, the team identified significant upregulation in the expression of 14 genes in brain tissue with ADHD compared with control brain tissue. For example, they saw differences in gene expression for the neurotransmitter glutamate, which signals between brain cells and is important for attention and learning.
The authors note that the findings show ADHD shares similarities with autism, and other conditions, and may reflect differences in how the brain functions. (NIH authors: G. Sudre, D.E. Gildea, G.G. Shastri, W. Sharp, B. Jung, Q. Xu, P.K. Auluck, L. Elnitski, A.D. Baxevanis, S. Marenco and P. Shaw, Mol Psychiatry 2022; DOI:10.1038/s41380-022-01844-9)
NINDS: MOUSE STUDY EXPLORES HOW BRAIN ACTIVITY IS FINE-TUNED
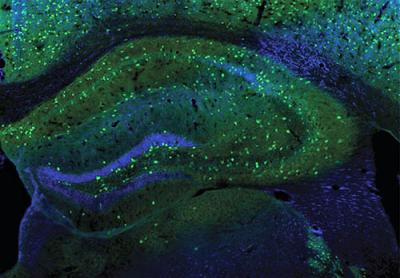
CREDIT: W. LU LAB, NINDS
NINDS: NINDS scientists revealed a new daily pattern in inhibitory synapses related to the sleep-wake cycle. Shown: Interneurons (green) in a mouse hippocampus, which play a subtle but powerful role in balancing neural activity during the sleep-wake cycle.
When our brains aren’t being flooded with new information, connections known as inhibitory synapses dampen neural activity—an essential step in consolidating information and forming memories during sleep. Using a mouse model, NINDS scientists revealed a new daily pattern in inhibitory synapses related to the sleep-wake cycle.
The researchers recorded electrical signals in the hippocampus, a part of the brain that supports memory formation, to examine inhibitory synapse activity during sleep and wakefulness. During wakefulness, tonic (steady) inhibitory activity increased, while phasic (fast) inhibitory activity decreased. In addition, awake mice also showed a higher enhancement of the activity-dependent inhibitory response, hinting that inhibitory synapses might be strengthened more by wakefulness than sleep.
Further experiments showed that synaptic changes in awake mice resulted from an increased number of gamma-aminobutyric acid type A (GABAA) receptors. GABA is an inhibitory neurotransmitter that suppresses neural activity. Blocking GABAA receptors reduced synaptic changes, suggesting that the accumulation of these receptors during wakefulness may contribute to greater inhibitory synaptic plasticity, a critical factor in learning and memory.
The investigators then examined whether parvalbumin or somatostatin, two types of inhibitory interneurons believed to regulate synaptic changes in the hippocampus, were responsible for the increased plasticity. Applying optogenetics, a technique using light to manipulate cell activity, researchers found that parvalbumin, but not somatostatin, interneurons contributed to more GABAA receptors and stronger connections in awake mice.
“Inhibition is actually quite powerful because it allows the brain to perform in a fine-tuned manner, which essentially underlies all cognition,” said NINDS Senior Investigator Wei Lu. The new findings could help researchers understand the human sleep-wake cycle and memory, as well as abnormal brain rhythms in neurological disorders, such as epilepsy. (NIH authors: K. Wu, W. Han, and W. Lu, PLoS Biol 20:e3001812, 2022)
NCI, NIAID: SOME CANCER AND AUTOIMMUNITY DRUGS MAY INCREASE SUSCEPTIBILITY TO SARS-COV-2 INFECTION
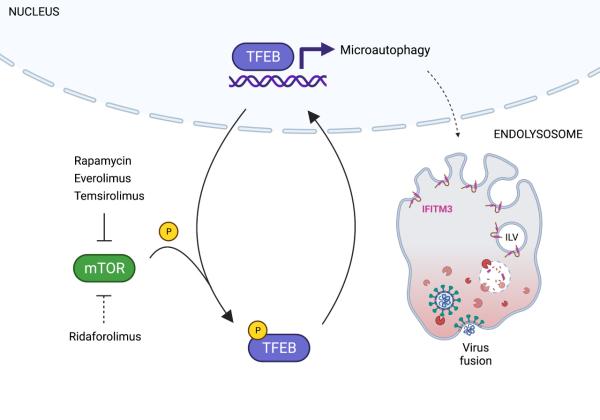
CREDIT: NCI
A research team led by Guoli (Scarlett) Shi and Alex Compton of NCI has defined a new mechanism by which a class of FDA-approved drugs known as mTOR inhibitors causes immunosuppression and could make people more susceptible to infection by respiratory viruses such as SARS-CoV-2 and influenza A. Those drugs include rapamycin and its derivatives (rapalogs) and are used to treat people with cancer and autoimmune conditions.
Traditionally, mTOR inhibitors are thought to cause immunosuppression by inhibiting the body’s infection-fighting T cells. But the scientists discovered that rapamycin also degrades antiviral membrane proteins inside of a cell, disarming the cell’s intrinsic defenses against pathogen invasion.
Using mouse and hamster models of infection, the investigators found that exposure to rapalogs enhanced SARS-CoV-2 titers in lungs and exacerbated COVID-19 severity compared with control animals. Further experiments on primary human respiratory cells showed that rapalogs made those cells more susceptible to SARS-CoV-2 infection, at least in part by disabling intrinsic immunity and facilitating virus entry.
Moreover, the research team identified a less potent rapalog—ridaforolimus—that had no significant effect on susceptibility to viral infection or disease severity. “We can use this variant of rapamycin as a template for the development of next-generation mTOR inhibitors that inhibit cancer cell proliferation without limiting a person’s defenses against virus infection,” Compton said. (NIH authors: G. Shi, A.I. Chiramel, T. Li, K.K. Lai, S. Majdoul, T. Dempsey, P.A. Beare, J.W. Yewdell, S.M. Best, and A.A. Compton, J Clin Invest 132:e160766, 2022; DOI:10.1172/JCI160766)
This page was last updated on Sunday, January 8, 2023