The Intersection of Man and Machine
Bionics Gives New Hope to Those Living With Physical Disabilities
If popular culture is any indication, the notion that bionic technology will someday redefine the boundaries of human function has long held our collective fascination. “We can rebuild him; we have the technology,” began the 1970s classic television series The Six Million Dollar Man. Massachusetts Institute of Technology (MIT) Professor Hugh Herr has the technology and is building bionic limbs to end physical disability caused by trauma and disease. Herr heads the Biomechatronics Research Group at MIT’s Media Lab (Cambridge, Massachusetts) and co-leads the MIT Yang Center for Bionics. He shared his story, “On the Design of Bionic Limbs: The Science of Tissue-Synthetic Interface,” at a recent Wednesday Afternoon Lecture Series (WALS) presentation.
A Life Refocused
As a young man Herr was a rising rock-climbing talent, establishing himself as one of the sport’s best. But in January 1982, an ice climb on New Hampshire’s Mount Washington altered his trajectory: Herr lost both legs below the knee to frostbite after he and his partner became disoriented in a blizzard and spent four harrowing days stranded on the mountain.
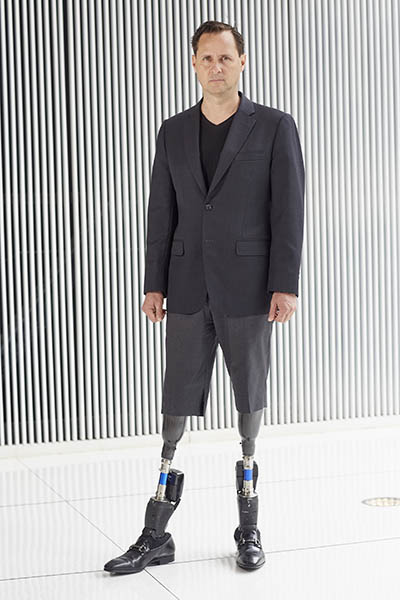
CREDIT: MATTHEW SEPTIMUS, MIT
MIT Professor Hugh Herr, who designed his own prosthetics after he lost both legs in a climbing accident, shared his story at a WALS lecture at NIH recently.
A set of standard prosthetic limbs enabled Herr to walk again, but he was unsatisfied with the rough design and insistent on resuming his climbing career. Herr took to the machine shop and fabricated his own legs that were optimized for the vertical world: Specialized attachments enabled him to adjust his height from five- to nine-feet tall, or balance on razor-thin rock fissures and vertical ice walls. He was able to climb at a more advanced level than ever before. “This was a tremendous lesson for me,” said Herr. “Technology has the ability to heal and rehabilitate, and in my own case, extend human capability beyond normal physiological levels.”
The Bionic Age
In 2002 Herr went on to invent the world’s first powered ankle-foot prosthesis. Known today as the Empower, the device has been fitted to more than 3,000 people who’ve had lower-extremity amputations. The computer-controlled robotic ankle restores natural gait through a motor, three processors, and 12 sensors. Herr uses the technology himself but saw room for improvement. “I felt like I was the backseat driver in a very powerful car,” he said. So, his MIT team set to work linking the nervous system directly to the electromechanics and allowing the device to release feedback to the central nervous system (CNS)—a concept he calls “neuro-embodied design.” In order to take full advantage of the sophisticated prosthesis, however, they first needed a new amputation paradigm.
Herr refers to the surgery performed on him as a “Civil War–era amputation.” While surgical techniques have advanced, amputations today still tack down muscles in a static position. This disrupts the person’s awareness of their limb’s position and movement, a sense known as proprioception: Joints typically move by an interplay between agonist and antagonist muscle pairs. The agonist contracts, stretching the antagonist and activating natural sensors embedded in tendons, muscles, and skin that inform the brain on the limb’s force, position, and speed.
In 2014, Herr led his MIT group in the invention and preclinical development of the agonist-antagonist myoneural interface (AMI) amputation technique. This procedure surgically reconnects natural muscle pairs, or reconstructs new ones, restoring the dynamic link that provides critical proprioceptive feedback. Surface electrodes placed over each AMI pair can then translate voluntary movement (or a computer-generated electrical signal) to a bionic prosthesis, allowing the user to control their new limb.
In 2016, bolstered by this foundational preclinical research from MIT, Brigham and Women’s Hospital (BWH) performed a first-in-human amputation using an AMI surgical design under the direction of Matthew Carty, director of the Lower Extremity Reconstruction Program. The first person to receive the AMI procedure was Jim Ewing, who had his left ankle amputated after a climbing accident in the Cayman Islands. Herr designed a robotic ankle with two planes of motion that connected to Ewing’s AMI muscle pairs. The results were remarkable. “Jim showed natural mechanics mediated through the electromechanics on steps and slopes, the same involuntary movements that happen in intact biological limbs,” said Herr in his talk. In this preliminary study, Ewing’s CNS was able to exert both voluntary and involuntary control over his bionic ankle. Subsequent studies on a larger population of persons with an AMI amputation have shown that even when using traditional prostheses, AMI recipients experienced less pain and greater perception of their phantom limb. The MIT team, in collaboration with Carty at BWH are now applying the procedure to the upper extremities, too.
Thirty patients have now received the AMI surgical procedure primarily at BWH. In addition, the Walter Reed National Military Medical Center (Bethesda, Maryland) has recruited patients and performed the surgery as well. In order for more people to have access to such procedures and bionic limbs, Herr and his colleagues have been advocating for insurance reimbursement. “The soft-tissue surgical work performed during the AMI procedure has been largely covered by insurance,” Herr said. The bionic hardware itself is not covered yet and Herr hopes that one day it will be covered, too.
Preclinical studies underway at the MIT lab are exploring ways to create seamless bidirectional communication between bionic prostheses and humans, a concept Herr refers to as “closing the loop.” Small magnets are being implanted into AMI muscles to precisely track contractions. This technology, called magnetomicrometry, will one day enable the bionic joint to respond in real-time to AMI muscle contractions.
Another neural interfacing technology Herr is exploring is optogenetics. Proprioceptive feedback on prosthetic joint position and force is sent back to the nervous system by optogenetic stimulation: This technology genetically alters neurons in the nerve that innervates muscle to respond to light. The computer in the prosthesis can then activate light emitters to stimulate AMI muscles, effectively letting the brain know exactly how the artificial joint is moving.
Preclinical data collected by Herr’s lab has also shown that skin cells grafted to muscle could potentially restore the sense of touch through sensors on the bionic limb. To create a wired, percutaneous connection between the peripheral nervous system and the bionic limb, Herr’s lab is using a technique called osseointegration: A titanium rod affixed to the bone bears weight and runs wires from internally implanted electronics directly to the prosthesis. Two patients are currently enrolled in a clinical trial funded by the Defense Advanced Research Projects Agency, and have undergone the procedure at the above-knee amputation level.
Herr ended his talk with dramatic drone footage of Jim Ewing returning to the site of his accident to ascend a seaside cliff using a climbing-specific ankle-foot prosthesis. “The focus of society has to be...to provide people the freedom of movement, the freedom of sensory experience,” Herr said as he concluded his talk. “My hope and life’s mission is to provide people with the freedoms that they don’t currently have.”
From hearing aids to artificial heart valves, many of us are already, in a sense, bionic. We may not be leaping over skyscrapers (yet), but new technology has had a profound impact on the lives of those with physical disability, and is rapidly filling the void between our actual and potential selves.
To view the May 5, 2021, videocast of Hugh Herr’s WALS lecture “On the Design of Bionic Limbs: The Science of Tissue-Synthetic Interface,” go to https://videocast.nih.gov/watch=41586.
NIH’s Work Helping People With Movement Disorders
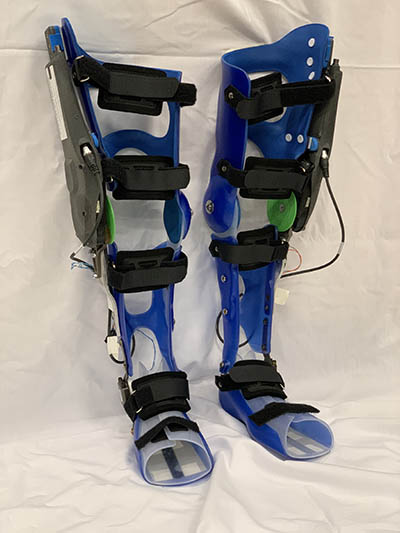
CREDIT: THOMAS BULEA, CC
This NIH pediatric exoskeleton is for children with cerebral palsy and other movement disorders. The device uses custom actuators from Agilik developed as part of a cooperative research and development agreement with NIH, along with embedded sensors and microcontrollers, to provide overground gait training while worn.
NIH is also investigating how technology can improve the function of people living with movement disabilities, not necessarily from amputation, but as a result of neurologic disorders such as cerebral palsy (CP) and spina bifida. Diane Damiano, chief of the NIH Clinical Center’s Functional and Applied Biomechanics (FAB) Section has assembled a multidisciplinary team of scientists and engineers, who’ve been developing wearable robotics and using brain-imaging technologies to assess and train motor coordination and tailor rehabilitation interventions. Much of Damiano’s work has focused on children with CP, a condition caused by a brain injury or malformation that occurs before, during, or after birth. CP affects a person’s ability to perform motor skills, including walking. FAB researchers found that two children with CP might use completely different parts of their brain to perform such a skill, suggesting that any training strategy harnessing the brain’s ability to rewire itself—a concept known as neuroplasticity—should be fit to the individual.
In 2017, the FAB team unveiled the first prototype of a powered lower-limb exoskeleton that improved upright posture and walking speed in children with CP. The wearable device uses external sensors and motors to facilitate the child’s natural effort and has evolved in recent years. “We expanded the mechanical design and software to provide multiple modes of assistance and identify the most effective one for each individual,” said lead investigator Thomas Bulea.
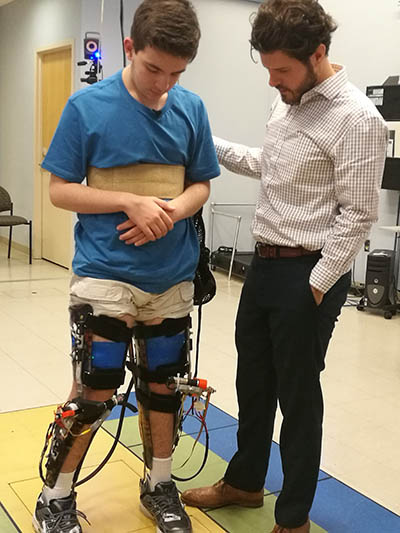
CREDIT: NIH CLINICAL CENTER
Alexander Theodorakos, a participant in a research protocol at the NIH Clinical Center evaluating a new pediatric exoskeleton for treatment of crouch gait, and study leader Thomas Bulea discuss how the device changes the way the legs move while walking.
FAB recently partnered with Vancouver, Canada–based Agilik Technologies Inc. to update the original exoskeleton into a streamlined, lightweight, wireless unit. The exoskeleton can also be outfitted with electric stimulators to cue key muscles involved in knee extension during walking, a technique that has begun to show promise. Bulea hopes the technology—combined with FAB’s responsive software—can eventually be made widely available to patients.
To determine the effectiveness of the exoskeleton, researchers use electroencephalography (EEG) to record brain activity, electromyography (EMG) to measure muscle response to nerve stimulation, and motion-capture techniques to record movements. This information can be incorporated into personalized software and robotic interventions. “Our software takes information from sensors at 100 times per second on limb position and velocity and deduces what the person is trying to do in real time,” said Bulea. “So the robot adjusts to what the person wants to do.” His team has begun studies on patients with spina bifida (a birth defect that occurs when the spine and spinal cord don’t form properly) and are planning to expand the technology to other conditions such as stroke and incomplete spinal cord injury.
Damiano’s current focus is on using new technologies to intervene during the earliest stages of motor development. Her group worked with Ashburn, Virginia–based Aretech, Inc. to modify the company’s ZeroG computerized harness system that partially supports a person’s weight. The miniaturized design enables infants with CP to learn to move more easily. “If kids are born and it takes extra effort to move much [due to CP] they often stop trying as hard,” said Damiano. “They may even lose brain pathways needed to perform certain coordinated movements in the future.” A pilot study on infants with CP older than 1 year of age found that using the harness system significantly improved mobility skills compared with a control group. Damiano hopes to apply this technology to babies with CP. “They have the most capability,” she said, pointing to recent success in animal studies. “There’s a brief window of time from 3 to 6 months of age where they may be able to recover [from] the brain injury.”
FAB is set to begin a new neurofeedback protocol. They designed a computer interface in which the patient practices a movement (such as flexing the ankle) while receiving EEG and EMG feedback on what part of their brain they are using. Tactile cues such as resistance or electrical stimulation can then push the patient to recruit different parts of their brain that better control the movement. “We aim to do individualized training to promote use of the injured side [of the brain] and improve muscle activity,” said Damiano.
FAB continues to discover ways to help people with all kinds of movement disabilities by bringing new interventions to the rehabilitation arena. “We’re trying to expand the use of technology to train people to do more on their own—ultimately without devices—not merely to replace what they can’t do,” said Damiano. “To do this better, we need to develop ever smarter rehabilitation technology.”
The FAB is a multidisciplinary group of scientists, staff, and trainees. FAB is charged with evaluating patients with complex motor disabilities; providing biomechanical expertise and support to other Clinical Center protocols; conducting innovative research; establishing productive scientific collaborations with other scientists at the NIH, academic institutions, and industry, nationally and internationally; and training the next generation of rehabilitation researchers. For more information, go to https://clinicalcenter.nih.gov/rmd/fab/index.html.
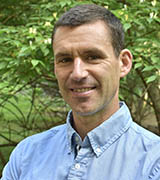
Michael Tabasko is a science writer-editor for The NIH Catalyst.
This page was last updated on Tuesday, February 1, 2022