Piecing Together the Autism Puzzle
NIH Researchers Tackling the Problem on All Fronts
Dan Hall’s son was two years and nine months old when he was diagnosed with an autism spectrum disorder (ASD). “He was very verbal, then all of a sudden he stopped talking. We thought he was deaf,” said Hall. “Back then people didn’t really know what autism was, so it wasn’t our first thought.” That was 12 years ago. Hall has learned plenty about autism since then. Today he’s the manager of NIH’s National Database for Autism Research (NDAR), which consolidates autism biomedical data on 25,000 participants and makes it available to qualified researchers. “Data sharing really provides the opportunity to uncover and accelerate scientific research,” said Hall. “Data aggregation is needed to help scientists uncover the patterns of what may be many autisms.” He expects the NDAR database to grow to contain data on 100,000 participants within the next four years.
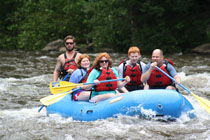
Dan Hall (right), who’s the manager of NIH’s National Database for Autism Research and has a teenage son with ASD, enjoys rafting on the Big Pigeon River (Hartford, Tenn.) with his family.
And so goes one of many NIH projects on autism, first described by psychiatrist Leo Kanner in 1943 as “the innate inability of certain children to relate to other people.” ASDs—which include autistic disorder, Asperger syndrome (autism-like problems in social interaction and communication but normal intelligence and verbal skills), and “pervasive developmental disorder–not otherwise specified” (PDD-NOS), the disorder Hall’s son has—are neurodevelopmental disabilities characterized by impairments in social interaction and communication and restricted, repetitive, and stereotyped patterns of behavior. Symptoms typically become apparent before the age of three. Although there is not yet a cure for ASD, there are ways to minimize symptoms and maximize learning.
More than one million teens and children in the United States are affected by an ASD. According to the March 30, 2012, issue of CDC’s Morbidity and Mortality Weekly Report, an estimated one in 88 American children has been identified with an ASD, and it is almost five times as common among boys (1 in 54) as among girls (1 in 252). This estimate marks a 78 percent increase since 2007, when the CDC reported that about one in 150 children had an ASD. Some of the increase is due to the way children are identified, diagnosed, and served in their local communities, although exactly how much is due to these factors is unknown.
No one knows the exact cause of ASD, but scientists do think that both genes and environment play important roles. Although hundreds of genes are associated with ASDs, less than five percent of the autisms are single-gene disorders. Most ASDs are the result of rare genetic variants, and many are associated with genes for proteins that are involved in the formation or strengthening of synapses.
NIH has conducted and supported ASD research for more than 20 years. Although the National Institure of Mental Health (NIMH) is the lead institute for autism research—and the largest single source of funding for autism research in the United States—other institutes are doing autism research as well. Here we highlight a few of the NIH intramural scientists who are helping to assemble the autism puzzle.
ASD Clinical Research Team
One of NIH’s distinguished autism researchers is senior investigator Susan Swedo (NIMH) who also studies childhood obsessive-compulsive disorder (OCD). In 1998, she led a NIMH team that discovered how a strep infection could trigger the abrupt onset of an OCD known as PANDAS (Pediatric Autoimmune Neuropsychiatric Disorders Associated with Streptococcal infections). In 2006, she helped develop the NDAR and established NIMH’s Pediatrics and Developmental Neuroscience Branch, one of only three U.S. multidisciplinary clinical-research teams dedicated to studies of ASDs. The team includes pediatricians, clinical psychologists, psychiatrists, neurologists, and speech pathologists. “One of the huge strengths of a fully integrated program such as this is our diverse training and experiences, which allow us to focus on different aspects of autism,” said Swedo. “Each of us sees something the others don’t.”
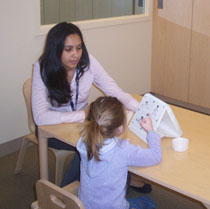
Lindsay Wood, NIMH
NIMH has one of only three U.S. multidisciplinary clinical-research teams dedicated to the study of autism spectrum disorders. Here, Lisa Joseph is conducting a behavioral test with a child who is part of a clinical trial.
ASD is thought to consist of several similar overlapping disorders. The problem, said Swedo, is that “ASD is studied as a single disorder by most groups.”
“Our goals are to get to meaningful subtypes of autism that link phenotype to genotype and etiology [and] lead toward new treatments that are efficacious and effective,” said team member Audrey Thurm, a clinical psychologist who supervises the screening protocol for the branch.
Swedo’s team is conducting a longitudinal study of at-risk toddlers (ones who already show signs of an ASD) to identify early predictors of the disorders. The researchers plan to identify connections between biological and behavioral markers and communication abnormalities.
Swedo and the team’s pediatric neurologist, Ashura Buckley, are investigating how abnormal sleep patterns may contribute to ASD. They found that some children with autism have deficits in rapid-eye-movement (REM) sleep, which is the dreaming cycle, as well as differences in non-REM sleep, particularly in the deep or slow-wave cycle. “Since the absence of REM sleep has been associated with problems in memory consolidation, it is tempting to speculate that the REM deficits are associated with failure to learn the complexities of social interactions,” said Swedo.
Buckley recently found that donepezil, which increases REM sleep in adults with Alzheimer disease, had the same effect in children with ASD who also have a REM deficit. The next step will be a full-scale clinical trial that Swedo will likely do in association with extramural collaborators. In addition, Thurm, Swedo, and Buckley will be looking at sleep and magnetic-resonance imaging (MRI) markers in toddlers who are at risk for autism.
Clinical Genomics
Among Swedo’s collaborators is senior investigator Owen Rennert in the National Institute of Child Health and Human Development (NICHD).
“Swedo’s group does the clinical phenotyping, and those diagnosed with ASD then come to us,” he said.
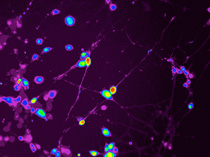
Dalton (Xiaozhuo) Liu, NICHD
Owen Rennert (NICHD) is investigating the molecular and cellular changes that occur in the developing autistic brain. He created these neuronal cells in culture by reprogramming skin cells from ASD patients.
Rennert is investigating the molecular and cellular changes that occur in the autistic brain during development. To study ASD neuronal cells in culture, he reprograms skin cells from Swedo’s ASD patients to form stem-like cells, which he coaxes to develop into neuronal cells. He then works with a cell-separation group at the National Institute of Neurological Disorders and Stroke to isolate the neurons from other neural cells and with outside researchers to analyze neuronal activity. Rennert predicts that they’ll find abnormal signaling in the ASD neurons.
If the prediction holds, then Rennert plans to identify the genetic pathways affected in ASD. “There are over 300 genes identified as being involved so far,” he said. “Therefore, it is likely that mutations in a host of rare alleles give rise to ASD and that they all contribute a very small percentage to the overall prevalence.”
Scanning the Brain
While Rennert approaches altered neuronal connectivity in autistic patients at a cellular level, senior investigator Alex Martin (NIMH) uses MRI to look at connectivity in the brain as a whole. In collaboration with the Children’s National Medical Center (Washington, D.C.), Martin and NIMH staff scientist Stephen Gotts use MRI scans to simultaneously view different regions in the brain and determine how different areas of the brain “talk” to one another. Martin is interested in a broad set of interconnected regions that make up the so-called social brain. “In the normal brain, this suite of areas is strongly interconnected and is composed of three minicircuits,” he said. “What we don’t know is what has gone wrong in the circuitry in ASD. Some circuits could be misfiring; others could be talking to each other too strongly or too weakly.”
By comparing MRI data from high-functioning adolescent males with ASD to matched adolescent males developing normally, Martin showed that communication between these circuits, rather than within an individual circuit, is impaired. “In patients with autism, the degree of connectivity varies with the degree of social activity impairment,” Martin said. He plans to do intervention studies in an attempt to “increase social communication abilities … to see if we can influence, using the intervention, the pattern of abnormal correlation,” he said. “Basically, we are looking at biological markers of the intervention.”
Martin is also using imaging techniques to view differences in the overall brain structure in autistic patients. Scientists have found that the prefrontal cortex of young children with ASDs is larger than that of children without an ASD. Gregory Wallace (NIMH), a research fellow in Martin’s lab, collaborated with senior investigator Jay Giedd (NIMH) to confirm a hypothesis that, in ASD, early overgrowth of neurons in the cerebral cortex may be followed by prematurely arrested growth. They used MRI scans to compare cortical thickness in high-functioning male adolescents with ASD to that of typically developing males. The MRI data indicated that for the ASD group, significantly thinner cortex surrounded the left temporal and parietal cortical regions. A follow-up study in the same patients found age-related thinning of the cortex in the ASD group but not in the typically developing group.
Environmental Toxins?
Another researcher exploring the relationship between abnormal cortical architecture and ASD is senior investigator Serena Dudek at the National Institute of Environmental Health Sciences (NIEHS). She wants to know how environmental factors play a role in brain development and how synaptic plasticity (the process by which the brain adapts to changes in its internal and external environment) during postnatal development is different from plasticity in the adult. She focuses on the nerve-signaling phenomena underlying synaptic plasticity—long-term potentiation (LTP), which strengthens synapses, and long-term depression (LTD), which weakens synapses and results in synaptic pruning. “Any gene mutation or environmental toxin that influences synaptic transmission would [modulate] the likelihood of getting LTP or LTD during development,” she explained. When such alterations occur during critical periods in development, there could be consequences for cognition with implications for ASD.
Dudek believes that the cortical overgrowth observed in ASD patients may be due to alterations in normal synapse pruning. As the brain develops through childhood it grows rapidly and the number of synapses per cerebral cortex neuron rises from about 2,500 in infancy to about 15,000—twice the number in the adult brain—by age three. As the brain matures, the synapses are pruned to get rid of the weaker connections and keep the strong ones. When the pruning process goes awry, then brain circuitry fails to develop properly.
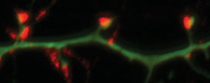
Maile Henson, NIEHS
Serena Dudek (NIEHS) wants to know how environmental factors play a role in brain development and the normal synapse pruning process, which goes awry in ASD. Pictured: synapses on cortical neurons in culture.
Dudek proposes that in ASDs, there is a reduction in cortical pruning during early childhood, resulting in the early surge in brain volume. “We are trying to establish what exactly are the molecular processes behind synapse activity-dependent pruning.” She believes that the pruning is regulated by the activity of different genes and could also be influenced by environmental toxins. But so far, no specific toxins have been identified as contributing to ASD.
Dudek and senior investigator Scott Young (NIMH), with whom she is collaborating, may have uncovered another piece of the autism puzzle. They are trying to identify molecular mechanisms that regulate synaptic plasticity in the relatively unexplored CA2 region of the hippocampus, an area of the brain that plays a role in memory formation and spatial navigation.
Young has shown that the number of vasopressin 1b receptors, which are important for aggressive behaviors, is very high in the CA2 region. His group created knockout mice without those receptors; the mice showed impaired social-recognition memory and less aggression. Dudek found that both vasopressin and oxytocin can enhance synaptic responses in the CA2. “We think [CA2] is going to turn out to be a missing piece of the autism puzzle,” she said. “There are a number of ASD-linked genes—and many neuromodulators and growth factors and their receptors—that are enriched in CA2.”
Hundreds of Genes
Senior investigator Jacqueline Crawley (NIMH) began using mouse models in the 1990s to examine some of the hundreds of genes that are implicated in ASDs. Her lab developed now widely used methods to measure ASD-associated behaviors in a strain of mice that normally display autism-like behaviors such as low sociability, low number of vocalizations in social settings, and large amount of time spent in repetitive self-grooming.
Recently, she and Jill Silverman (NIMH) published new data showing that an experimental compound, GRN-529, boosts social interactions and lessened repetitive self-grooming behavior in the mice. Crawley’s team followed up on clues from earlier findings hinting that inhibitors of the receptor, mGluR5, might reduce ASD symptoms. [Sci Transl Med DOI: 10.1126/scitranslmed.3004017 (2012)]
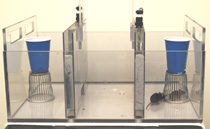
Mu Yang, NIMH
NIMH researchers recently found that in an autistic breed of mouse, an experimental compound increased social interactions and lessened repetitive grooming behaviors. After receiving the compound, an autistic mouse is trying to socialize with another (in the cage).
GRN-529 is part of a class of agents that inhibit activity of a subtype of receptor protein on brain cells for the chemical messenger glutamate, which are being tested in patients with an autism-related syndrome. Although mouse brain findings often don’t translate to humans, the fact that these agents are already in clinical trials for an overlapping condition strengthens the case for relevance, according to the researchers.
“Our findings suggest a strategy for developing a single treatment that could target multiple diagnostic symptoms,” Crawley said.
Still, there is no overall cure for ASDs.
“Right now the interventions are behavioral,” said Crawley. “They might get the child over the worst of the problems that may prevent . . . having meaningful social interactions, holding down a job, or living independently, but they don’t cure the disorder.” She hopes, however, that intervention at an early enough age could limit the impact of the symptoms and present “a real shot at a cure for autism.”
And scientists might have another shot at developing a cure if they can learn more about the genetic mutations that affect synaptic proteins.
“Synapses are forming and weakening all of the time; it’s a constant process,” said Crawley. “There is tremendous interest right now in what each of those single gene mutations do, if they have common downstream mechanisms, and what drugs might act on them.”
One researcher who knows a lot about the role of synaptic proteins in ASD is staff scientist Andy Mitz (NIMH), who, like Dan Hall, has a child with an autism-like disorder. Mitz is interested in the gene SHANK3, which encodes synaptic proteins. Errors in SHANK3 result in the rare disorder his son has—Phelan-McDermid syndrome, characterized by severe physical and intellectual developmental delays, especially in speaking and communicating—and are associated with ASD.
“Fifty percent of those with Pheland-McDermid syndrome are on the autism spectrum,” said Mitz, who founded the SHANK3 journal club. “It is connected to autism at every single level.”
Insights
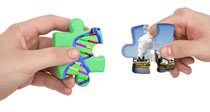
NIEHS
Incremental advances in brain research continue to fill in different parts of the autism puzzle, which is thought to include both genetic and environmental factors.
Gradual, incremental advances in brain research continue to provide insights into autism and other neurodevelopmental disorders. At NIH, autism research spans multiple institutes and disciplines, with each different approach filling in different parts of a complex neurological puzzle. As one NIH autism researcher put it, “the future of ASD will be one of personalized medicine—having a detailed multidimensional fingerprint of genetics and anatomy [that] overlaps with which interventions will be most effective.” NIEHS
This page was last updated on Monday, May 2, 2022