Starving Malaria in Red Blood Cells
Understanding How Parasite Feeds May Lead to New Treatments
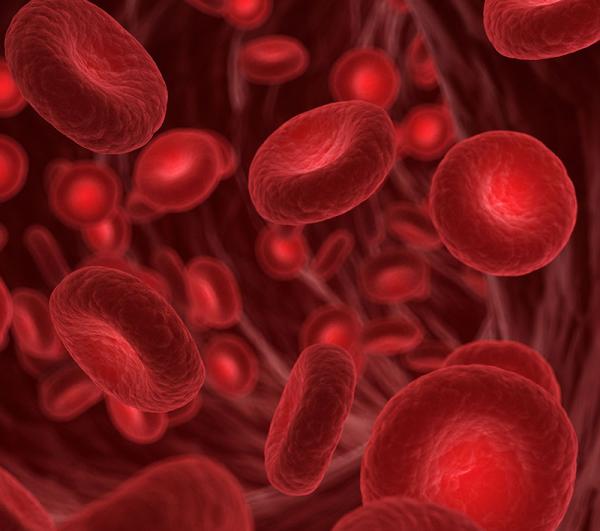
IRP researchers are attempting to develop new treatments for malaria that prevent vital nutrients and other materials from entering or exiting the red blood cells that malaria parasites hide inside.
World Malaria Day, commemorated annually on April 25, highlights the need to end an infectious disease that sickens nearly 250 million people around the world each year, killing more than 600,000 in regions where it is common. Children are particularly susceptible to its deadly effects.
Unfortunately, there is still no highly effective vaccine against malaria, so management is mostly limited to preventive measures like bed nets and medications that treat the infection, which must be taken over an extended period to effectively treat the disease and stop it from spreading. However, the speed with which the malaria parasite, Plasmodium falciparum, adapts to antimalarial drugs has created a critical need for novel treatments — a need that IRP researchers led by Joshua Zimmerberg, M.D., Ph.D., are taking a unique approach to filling.
Dr. Zimmerberg’s team is specifically using its expertise in cell biology to better understand how the malaria parasite survives inside the red blood cells it invades. An expert in how cell membranes are constructed and remodeled to meet the needs of our bodies, Dr. Zimmerberg believes structural changes the malaria parasite causes in those cells could be excellent targets for new types of drugs.
“Malaria causes a huge amount of devastation and a lot of child death, especially among children under 5 years old,” Dr. Zimmerberg says. “I felt I had something to contribute because of the large number of membranes that prominently change their identity and location within infected cells. This type of membrane remodeling seems to be important in malaria and other diseases.”
Red blood cells are oddballs when it comes to their structure. Unlike our other cells, which are filled with DNA and protein-making machinery, red blood cells are empty. This allows them to hold the hemoglobin necessary to carry oxygen to our organs, as well as change shapes to squish through narrow blood vessels and capillaries. Unfortunately, it also makes them an ideal space where the malaria parasite can settle and reproduce.
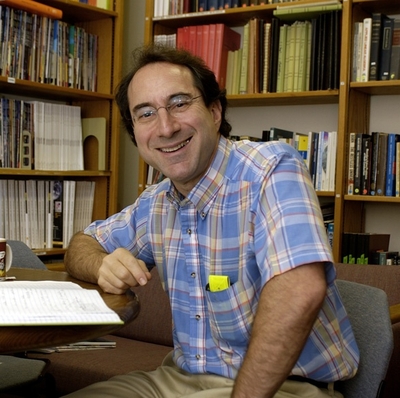
Dr. Joshua Zimmerberg
Dr. Zimmerberg and his colleagues are using a powerful electron microscope to explore the interior of the hijacked red blood cells and identify how nutrients pass through these barrier layers. His team is also able to take time-lapse movies using this technology, so they can watch the membranes transform in real time.
“Once the malaria parasite gets into the red blood cell, it takes over,” Dr. Zimmerberg explains. “It starts by wrapping itself in the red blood cell’s outer membrane, which it then pinches off, and the parasite becomes the cell’s new nucleus.”
The pinched-off cell becomes a protective sac called the parasitophorous vacuole. The malaria parasite can hide from the body’s immune system here, but it must still carry out all the typical functions of a living organism, including bringing in nutrients, expelling waste, and reproducing. To do this, it must find ways for the nutrients it needs and the waste it produces to cross not just its own cellular membranes, but also those of its host cell. That means setting up systems to transport molecules across membranes and building out small knobs along the blood cell’s outer membrane where parasitic daughter cells can be released into the bloodstream. Targeting any of these new structures may disrupt the life cycle of the parasite and stop the infection from spreading inside the body.
Dr. Zimmerberg and his colleagues — Mattias Garten, Ph.D., who is now at Stanford University, and Joshua Beck, Ph.D., now at Iowa State University — discovered that the distance between the outer membrane of the parasite and the protective sac it resides in varies. In some places, the two touched while in others there was a space at least 20 nanometers wide, and it turned out this arrangement was important. In the ‘sticky spots’ where the membranes came into contact, the team discovered molecules capable of transporting fat molecules, also known as lipids, through the barrier.1 This differed from the more open spaces, where scientists had previously found a certain variety of salt transporter.2
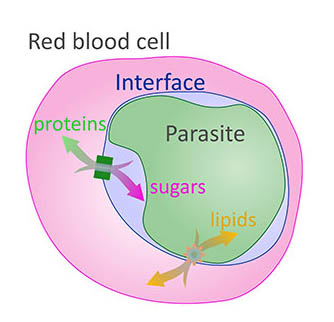
This diagram shows how nutrients must pass through multiple cellular membranes in order to reach the malaria parasite residing in a red blood cell.
“The parasite has been packaged in a very intelligent way,” Dr. Zimmerberg says. “It has certain proteins that are ready for release almost as soon as the red blood cell is taken over. These become pumps that move nutrients through channels in the membranes.”
Because many drugs are fat-soluble, the scientists’ discovery about where and how fats travel through the multiple membranes around the parasite may eventually lead to the development of antimalarial drugs that starve the parasite by disrupting its nutrient supply.
“This study came out very nicely and gave us a better understanding of how intimately the parasite cooperates with the host cell,” says Dr. Zimmerberg. “By the end of this whole story, it dawned on us that it wasn't fair to think of each membrane as being completely separate, but rather to see them working together as the interface controlled by the nucleus of the parasite. Through all of this prepackaging and engineering and planning, there's a whole plan that gets put into place to change the architecture of the red blood cell.”
There is still a lot to learn about the molecules involved in triggering the structural changes within an infected red blood cell, but Dr. Zimmerberg is optimistic that his studies can lead to helpful treatments.
“We can see the architecture, but we don’t know much about what kinds of proteins are creating it,” he says. “However, if we disrupt any of these steps, we will disrupt the whole system. And with malaria, because unique proteins are involved, they can be uniquely targeted.”
Subscribe to our weekly newsletter to stay up-to-date on the latest breakthroughs in the NIH Intramural Research Program.
References:
[1] Garten, M, Beck, JR, Roth R, Tenkova-Heuser, T, Heuser, J, Istvan, ES, Bleck, CKE, Goldberg, DE, Zimmerberg, J. Contacting domains segregate a lipid transporter from a solute transporter in the malarial host–parasite interface. Nat Commun. Jul 30, 2020;11(1):3825. doi: 10.1038/s41467-020-17506-9.
[2] Garten M, Nasamu AS, Niles JC, Zimmerberg J, Goldberg DE, Beck JR. EXP2 is a nutrient-permeable channel in the vacuolar membrane of Plasmodium and is essential for protein export via PTEX. Nat Microbiol. 2018;3(10):1090-1098.
Related Blog Posts
This page was last updated on Tuesday, May 23, 2023