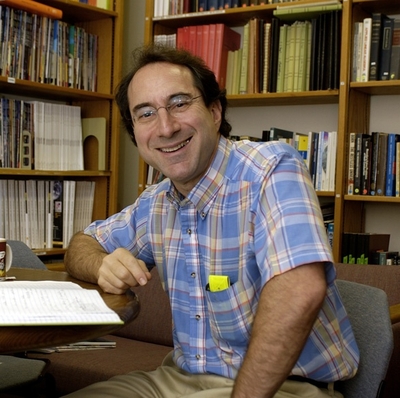
Joshua Jay Zimmerberg, M.D.,Ph.D.
Senior Investigator
Section on Integrative Biophysics
NICHD/DIR
Research Topics
Forty years of membrane remodeling
Fusion and fission are the essence of complex membrane dynamics in living cells. Fusion and fission are key elements of synapses and other dynamic cellular trafficking networks, the instants when organelles gain or lose their identities. Without fusion and fission, enveloped viruses and parasites could not enter cells, replicate, or exit cells; nor would inflammatory cells respond and kill such invaders, or deal with sick cells.
My earliest work concentrated on model membrane systems, the physical properties and theoretical pathways required for membrane fusion to occur and the discovery that tension spreads headgroups for hemifusion, then pulls open fusion pores to allow coalescence of adherent bilayers. However, model systems, while able to focus on basic membrane biophysical properties and help develop a theoretical framework to understanding membrane interactions, were a simplification that ignored the important roles of proteins. Including the role of proteins in these fundamental biophysical processes was both fruitful and informative culminating in what we believed to be a canonical framework for understanding both fusion and fission. I introduced a simple paradigm: proteins act as catalysts (bilayer topology-isomerases) lowering the huge energy barriers to membrane remodeling steps. A few amino acids of a specialized protein domain can reversibly enter the hydrophobic membrane matrix or cover the headgroups as inclusions or scaffolds, respectively, and transiently alter the thermodynamics of the system by specific protein-lipid interactions. By combining quantitative light microscopy with electrophysiology, and reconstitution of fusion and fission in lipid bilayer membranes, we constructed hypotheses with predicted fusion intermediates whose dimensions were deduced by continuum theory and fits to experiments. Since the predicted sizes were detectable by cryo-electron microscopy, we labored to achieve the highest resolution electron microscopy of hydrated membrane fusion events in order to understand how proteins catalyze the new configurations of lipids that ultimately mediate these processes. By successfully introducing to the NIH a new technology, the Volta Phase Plate, we managed to actually visualize for the fusion of the influenza virus (IFV) the predicted hemifusion diaphragm, and the measurements of its dimensions fit the predictions of continuum theory. But another result was unexpected: the fusion protein of IFV, hemagglutinin (HA) catalyzed the actual breakage of membranes, leading to free membrane edges – often in great profusion.
To understand how this was unexpected, we must consider the physical forces that act on lipids in solution. Membranes hate edges. The lipid bilayer is self-assembling because its free energy of cohesion (that comes in part from enthalpic attractive forces between hydrocarbon chains and in part from the entropic hydrophobic effect that minimizes interfacial area) automatically ensures stability of the lipid bilayer. Formally, the edge of an otherwise lamellar membrane has a large linear tension, i.e. should be a high energy region that the membrane seeks to minimize. Nevertheless, we observe that ‘free edges’ actually outnumber hemifusion diaphragms for certain lipid compositions of target membranes. Such edges only occur in close vicinity of activated HA molecules. indicating that they are triggered to form by the same event that triggers full fusion: namely, the amphipathic helix of HA being ejected from HA and binding to the target bilayer. We can only presume for the moment that the HA fusion-peptide somehow stabilizes the observed membrane edges, i.e. drastically lowers bilayer line-tension. Here we propose to test that hypothesis, and ask if changing lipid composition or membrane physical properties is a general biological process used to promote protein stabilized edges for the expressed purpose of introducing or preventing leakiness through membrane breaches in dynamic membrane transformations associated with IFV infection, the egress and invasion of malaria and toxoplasma parasites, and the loss of skeletal muscle enzymes in T-tubule reorganization of dystrophic animals and patients.
Personally, I am proud of my laboratory for these advances. A molecular understanding of complex events at the cell membrane requires the synergy of approaches and perspectives. I recruited a quality team of scientists, all of whom are able to work effectively on various aspects of fundamental membrane biology. Each person came with different expertise and interests, yet we all join together to study cell membranes with powerful biophysical techniques that are both cutting edge and quantitative. The best moments come one day each week, when we convene around a table for a whole afternoon, review our work and the literature, and face our scientific barriers to ferment new approaches. Our two-fold common focus is the biophysics of membranes in these living systems, and advancing the technology of quantitative imaging in bilayers, cells, tissues, and organisms including humans. As we learn each other’s language and science, impressive benefits have emerged in all of the problems we seek to solve, as the structure of viral infection, parasite life cycle, and intracellular muscle tubules all focus our biophysical thinking and deductions to the reality of the structures that membranes actually form in these living systems. Our ultimate goal is to test the new hypothesis we develop from synthesizing our biophysical predictions with our ultrastructural observations – namely, when we can test the hypothesis in living cells optimally imaged with the highest quality light microscope at exactly the right time and the right place.
Biography
Joshua Zimmerberg, M.D., Ph.D. trained in Developmental Enzymology, General Physiology, Membrane Biophysics and Neuroscience, and Cell Biology and early Development, with Olga Greengard, Alan Finkelstein, Adrian Parsegian, David Epel, and Daniel Mazia. More recently he trained in electron microscopy with Tom Reese. He builds teams of researchers to vigorously pursue the deeper understanding of membrane fusion, fission, phase, hydration, curvature, and poration as manifested in syncytia formation, cellular secretion, calcium homeostasis, collagen synthesis, viral infection, fertilization, organelle shape, protein translocation, channel architecture, toxoplasma entry, plasmodial egress, and adipose cell response to insulin. Having initiated studies on the long-_term deep tissue and dynamic single molecule microscopy of living cell membranes, he now focuses on membrane domains in viral assembly, viral entry into cells, lipid/protein interactions in pathological processes of HIV, apoptosis, malaria, sickle cell, insulin resistance, and traumatic brain injury. He is currently developing new viral-like particle models for the study of SARS-COV-2 pathogenesis, new mouse models for fragile sarcolemmal muscular dystrophies, and he is the PI of a clinical protocol to research biomarkers in subjects with these disorders. In NICHD, he is the Chief of the Section of Integrative Biophysics and a Senior Investigator. He recently fulfilled the maximum 8 year term as the Associate Scientific Director for the Division of Basic and Translational Biophysics, NICHD, the Division he founded. Prior to that, he founded and led both the Program in Physical Biology and the Laboratory of Cellular and Membrane Biophysics, NICHD, throughout their existence. In the NIH more generally, he is the Senior Advisor to the DDIR for Imaging and Biophysics. He is past and present Chairman of the NIH Assembly of Scientists, elected representative of his peers.
Joshua Zimmerberg was named the 2021 recipient of the Sir Bernard Katz Award for Excellence in Research on Membrane Fusion, Fission, and Traffic. Bestowed by the Biophysical Society the award is named for the 1970 co-recipient of the Nobel Prize in Medicine or Physiology who discovered that neurotransmitters are released from membrane bound vesicles and travel across synapses to relay messages across neurons. Zimmerberg was recognized for his contribution to the understanding of how cell membranes fuse together and separate in exocytosis—the process of how membrane-bound vesicles are released from the cell, and endocytosis—the process of how membranes envelop material to take it into the cell. During his career, Dr. Zimmerberg’s discoveries have led to insights into how viruses enter cells, cellular invasion by the toxoplasma parasite and to an understanding of how the malaria parasite enters the cell, commandeers its resources, evades the immune system and progresses through the various stages of its life cycle.
Selected Publications
- Petersen JD, Mekhedov E, Kaur S, Roberts DD, Zimmerberg J. Endothelial cells release microvesicles that harbour multivesicular bodies and secrete exosomes. J Extracell Biol. 2023;2(4):e79.
- Rice A, Haldar S, Wang E, Blank PS, Akimov SA, Galimzyanov TR, Pastor RW, Zimmerberg J. Planar aggregation of the influenza viral fusion peptide alters membrane structure and hydration, promoting poration. Nat Commun. 2022;13(1):7336.
- Petersen JD, Lu J, Fitzgerald W, Zhou F, Blank PS, Matthies D, Zimmerberg J. Unique Aggregation of Retroviral Particles Pseudotyped with the Delta Variant SARS-CoV-2 Spike Protein. Viruses. 2022;14(5).
- Garten M, Beck JR, Roth R, Tenkova-Heuser T, Heuser J, Istvan ES, Bleck CKE, Goldberg DE, Zimmerberg J. Contacting domains segregate a lipid transporter from a solute transporter in the malarial host-parasite interface. Nat Commun. 2020;11(1):3825.
- Chlanda P, Mekhedov E, Waters H, Schwartz CL, Fischer ER, Ryham RJ, Cohen FS, Blank PS, Zimmerberg J. The hemifusion structure induced by influenza virus haemagglutinin is determined by physical properties of the target membranes. Nat Microbiol. 2016;1(6):16050.
Related Scientific Focus Areas
Biomedical Engineering and Biophysics
View additional Principal Investigators in Biomedical Engineering and Biophysics
Microbiology and Infectious Diseases
View additional Principal Investigators in Microbiology and Infectious Diseases
This page was last updated on Tuesday, December 3, 2024