A Glycobiology Pioneer Uncovers the Secrets of Sugar
Profile: John A. Hanover, Ph.D.
In autumn 1944 the Nazis blocked food supplies to German-occupied western Netherlands, plunging much of the country into a famine known as the Dutch Hunger Winter. By the time World War II in Europe drew to a close in May 1945 and allied forces liberated the country, over 20,000 people had died and millions of others had suffered from the effects of starvation. The trauma would prove imprinted into Dutch genes. Decades later, people who were in utero during the famine experienced higher rates of diseases such as obesity and type 2 diabetes (T2D). They had inherited what some researchers call the “thrifty gene”: Their bodies were conserving energy, as if they were still experiencing famine conditions.
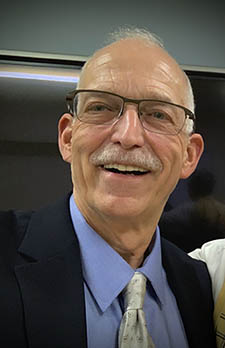
CREDIT: NIDDK
John Hanover
Scientists have since found that stressful environmental factors in the womb might alter the genetic traits of unborn offspring through methylation, an epigenetic process in which cells attach a methyl group to DNA. The addition of a methyl group can silence a gene, whereas removing it can boost a gene’s expression. Not only does this process affect a developing fetus, but it also acts on the fetus’s immature germ cells, which are precursors to egg and sperm. In the case of children born during the Hunger Winter, the methylation process likely led to insulin resistance and increased their risk—and that of their future offspring—to metabolic disease.
Exactly how cells sense environmental stresses and then signal molecular tools to reprogram DNA is not entirely clear. But understanding this concept might lead to new diagnostics and treatments for populations—such as Native Americans—at higher risk for metabolic diseases. John Hanover at the National Institute of Diabetes and Digestive and Kidney Diseases (NIDDK), who knows a thing or two about sugar, might have an answer.
Hanover is chief of NIDDK’s Laboratory of Cell and Molecular Biology (LCMB) and has dedicated nearly 40 years of research to glycoscience, the study of glycans. Glycans, also known as saccharides and carbohydrates, are sugar molecules that cloak the surface of all cells and festoon many proteins (glycoproteins), lipids (glycolipids), and other molecules, forming glycoconjugates. “We were focused on an area where there was very little existing knowledge,” said Hanover of his early years. Hiding in plain sight for decades, the function of these sugary appendages was largely unknown because of a lack of tools to study them. In the 1980s, Hanover and a handful of intrepid scientists began to unravel their secrets.
Fast forward to today and it turns out that when proteins or other molecules are tagged by certain glycans, it can signal big changes in cell-cell interactions, alter molecular traffic within a cell, and even allow a virus to recognize and infect a host. Scientists now know that glycans are inextricably linked to nearly every facet of cellular biology and implicated in conditions from cancer to neurodegenerative diseases and T2D.
For his outstanding contributions to the field of glycobiology, Hanover received the 2021 Karl Meyer Lectureship Award, the most prestigious international honor bestowed by the Society for Glycobiology each year. In a sense, Hanover’s career has come full circle. He joins prior recipients that include William Lennarz—who mentored Hanover as a graduate student at Johns Hopkins University School of Medicine (JHUSM, Baltimore)—and several scientists at NIH’s intramural research program who had an early influence on his career. The Karl Meyer award offers a pulpit to better advocate for a field that hasn’t been without challenge during an era in which flashier biomedical specialties such as genomics and epigenetics have often taken center stage. More recently, glycobiology has proven pivotal to discoveries such as antiviral therapies and our understanding of the immune system, and is finally finding its place within the tapestry of biomedical research.
Early influences
Growing up in Tulsa, Oklahoma, Hanover found a connection with the change of seasons and was drawn outdoors to the natural world. He had an insect collection and a small telescope to scan the stars, a hobby he continues today as an amateur astronomer. But it was in high school that a particularly outstanding biology teacher lit a career-commencing spark. “She just opened up my eyes to the joy of biology,” said Hanover in an oral history interview conducted by the Office of NIH History in 2009.
He graduated from the University of Tulsa (UT, Tulsa, Oklahoma) in 1976 with a master’s degree in chemistry and a bachelor’s in biology. It was somewhat of a different path than that of his parents. His mother worked for many years in a bank. His father was a jazz musician, a trombonist, who briefly attended college on a music scholarship. He never finished his studies and found a career as a butcher. Perhaps influenced by his father, Hanover himself is an amateur musician: He plays the flute, banjo, guitar, and trombone and loves performing Irish and bluegrass tunes.
During undergraduate studies at UT, he sought solid grounding in the physical sciences and was told he had a talent for teaching, a skill that would serve him well as a mentor and lecturer in the years to come. He gravitated toward research and began searching for graduate schools.
The next turning point was guided by a molecular biology professor at UT who had done a postdoctoral fellowship with Albert Lehninger, chair of the biochemistry department at JHUSM and author of Biochemistry, considered by many a seminal textbook. Hanover’s professor advised him that Lehninger’s department was the place to go to learn chemistry. Hanover was accepted to JHUSM’s graduate program and headed to Baltimore in 1976, a decision that would point him down the glycobiology road.
A foundation of mentors
While earning his Ph.D. at JHUSM, Hanover worked closely with Professor of Biochemistry William Lennarz, an impactful mentor whom he remembers fondly. “He ran his lab with incredible precision and brought the best out of everyone who ever worked for him,” said Hanover.
Lennarz had laid the groundwork for much of what is known about glycosylation, the process by which glycans attach to proteins or lipids across cell membranes. He supported Hanover’s dissertation on how glycoproteins assemble and arrange themselves on the cell membrane. And while it wasn’t popular at the time, they used model systems such as sea urchin embryos (Strongylocentrotus purpuratus) and yeast (Saccharomyces cerevisiae) to do chemistry alongside genetics and modern biology, which are all methods that the LCMB incorporates today.
Lennarz trained several notable scientists, including Gerald Hart, a friend and contemporary of Hanover’s. A few years later when Hart started his own lab he would use one of Hanover’s techniques to identify O-linked N-acetylglucosamine (O-GlcNAC), a simple form of glycosylation in which a monosaccharide is attached to a target protein. In the years to come the discovery would largely define both scientists’ careers. The O-GlcNAC modification began to show up as a major way for cells to signal changes in key pathways involved in cellular machinery and human disease.
In 1981, Hanover began a postdoctoral fellowship with Ira Pastan at the National Cancer Institute (NCI). Among other projects, the NCI lab was investigating how receptors on a cell’s surface bind to bioparticles such as hormones, proteins, and even viruses to allow entry into the cell, a process known as receptor-mediated endocytosis. It was the dawn of the molecular biology era and of learning to work with DNA. Hanover wanted to be a part of it. At NCI, he noted parallels to the Lennarz lab in the scientific rigor and creativity that Pastan was able draw from his trainees. During lab meetings Pastan would often challenge students to generate their own ideas of what the data meant, a mentoring approach that Hanover now uses with his own trainees to stimulate innovation.
By 1984, Hanover had found some scientific success, including a paper in Cell describing the kinetics of the protein clathrin as it encircles and moves molecules through a cell membrane, one of the early examples of how endocytosis works (Cell 39:283–293, 1984). In 1985, with Pastan’s support, he landed a job at NIDDK’s Laboratory of Biochemistry and Metabolism (LBM) and headed in a new direction.
The expanding role of glycans
Between 1986 and 1987, Hanover and his former colleague Hart published separate papers showing that O-GlcNAc occurred within a cell’s cytoplasm and on the nuclear pore complex (NPC), a semipermeable membrane barrier that regulates the flow of important proteins and genetic information between the nucleus and the rest of the cell. (Hanover’s paper: J Biol Chem 20:9887–9894, 1987; Hart’s paper: J Biol Chem 17:8049–8057, 1986) Their findings were controversial, almost heretical, and represented a paradigm shift that would not be widely accepted for many years. Prevailing dogma at the time said that glycosylation was restricted to the cell’s outer membrane, and the new discovery suggested that glycans might play a role in controlling access to the RNA factory sequestered inside the nucleus. At the time, the Hart and Hanover labs were the only ones using an enzyme-based assay trick that Hanover had developed in graduate school to visualize O-GlcNAc within the cell.
“It wasn’t a blinding insight, it was just the application of a new technology,” said Hanover, who would go on to characterize and clone the first nuclear pore glycoprotein.
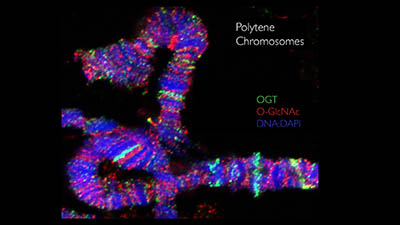
CREDIT: NIDDK
O-GlcNAc decorates chromosomes: O-GlcNAc is an abundant modification on chromosomes. Shown here is staining of O-GlcNAc transferase and O-GlcNAc on the giant chromosomes of Drosophila melanogaster.
From 1988 to 1990, his lab would also be the first to identify the enzymes that catalyzed the cycling (addition or removal) of O-GlcNAc to its protein targets, such as those guarding the NPC. Further work by Hanover and others would establish O-GlcNAc as critical in maintaining the stability of the NPC by regulating RNA assembly, transport, and translation. Improperly glycosylated proteins can dysregulate the NPC, which has been associated with DNA damage resulting in several diseases. Clinical trials today are exploring potential treatments for neurodegenerative conditions by targeting the enzymes that control O-GlcNAc cycling.
Hanover became chief of LBM’s Cell Biochemistry Section in 1991 and the chief of NIDDK’s Laboratory of Biochemistry and Biology in 1994. As technology advanced, glycobiologists began using tools such as mass spectrometry to identify new glycans and glycoconjugates. By the late 1990s, genomic sequencing led to discoveries revealing how glycans functioned in model organisms such as Caenorhabditis elegans and Drosophila melanogaster, opening the floodgates to new research possibilities. In 1997, Hanover’s postdoctoral fellow, William Lubas, was the first to isolate and clone O-GlcNAc-transferase (OGT) (J Biol Chem 14:9316–9324, 1997). The enzyme is found in abundance in the pancreas and responds to elevated blood glucose concentrations by boosting O-GlcNAc activity.
In 2010, Hanover became chief of LCMB, and ongoing research there has since linked the OGT glucose-sensing pathway to the development of T2D. Hanover credits that work to close collaboration with several talented scientists including Dona Love (NIAID), Michelle Bond (National Institute of General Medical Sciences, NIGMS), and Lara Abramowitz (NIDDK).
New technologies and new directions
So, if O-GlcNAc activity does indeed respond to environmental stresses such as hunger and diet, might it also play a role in reprogramming the DNA of future generations? Hanover’s team has been grappling with this question for nearly 15 years. They may have found the answer by using new enzyme-based tools that look at specific DNA methylation sites to prove that protein glycosylation influences the gene-expression pathway. “We see a dramatic change in DNA methylation if we block the cycling of O-GlcNAc in mice,” said Hanover of his lab’s new findings. “This link between transgenerational information transfer and nutrients, it’s sort of the Holy Grail of epigenetics.” The LCMB plans to submit their data for publication this year.
An advocate for glycobiology at NIH
When Hanover first came to NIH, the IRP was a “hotbed of glycobiology research,” he said. Elizabeth Neufeld, Victor Ginsburg, and Roscoe Brady had already made important contributions to our understanding of how carbohydrates play a role in cellular signaling and disease. “It was very nurturing,” said Hanover. “I think that that’s an important role of senior scientists, to maintain an environment that is conducive to the growth of young scientists.”
He found support from senior researchers such as Gilbert Ashwell and Vincent Hascall–both former Karl Meyer awardees (Stuart Kornfeld was another NIH recipient)—who would help him establish the Glycobiology Scientific Interest Group (SIG), which remains active today.
Now with nearly 300 published papers, Hanover remains highly involved as an advocate for the NIH intramural glycoscience community. He lectures both locally and internationally and was instrumental in developing a course at NIH’s Foundation for Advanced Education in the Sciences dedicated solely to glycoscience, one of the first in the country. He remains involved with several professional organizations and committees, such as an NIH Common Fund review panel, which serves to fund new tools to advance the field. In 2016, he cochaired the NIH Glycoday (now Glycosciences Research Day) that brought intramural scientists together with like-minded scientists from across the country and world.
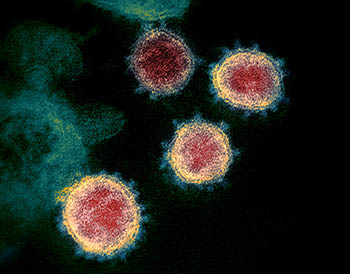
CREDIT: NIAID
Spike proteins (blue) crown SARS-CoV-2, the virus that causes COVID-19. Once the virus enters humans, the spike protein is decorated with sugars that attach to some of its amino acids, forming O-glycans. Loss of key O-glycans may facilitate viral spread to human cells.
“Many of his ideas have helped to advance glycobiology right here, creating a very vibrant community within the intramural program,” said Kelly Ten Hagen (National Institute of Dental and Craniofacial Research, NIDCR), who serves with Hanover as the Society for Glycobiology’s representatives to the International Glycoconjugate Organization. Ten Hagen represents a new batch of investigators at NIH who are pushing the boundaries of glycoscience research. Her recent collaboration with NIH Acting Director Lawrence Tabak (NIDCR) resulted in a study that found glycosylation of the SARS-CoV-2 spike protein influences how the virus recognizes and infects human cells (Proc Natl Acad Sci USA 118:e2109905118, 2021)
Today, Hanover takes great pride in mentoring the next generation of glycobiology researchers. Many of his trainees have gone on to pursue successful independent research programs uncovering how glycan-mediated pathways are involved in everything from cancer to immune deficiencies and the differentiation of stem cells. He advises his students to keep their eyes on the broader goals of their work when encountering the inevitable setbacks and successes of tackling a research problem. “Persistence is the key thing,” said Hanover, drawing from his own experience pursuing projects that weren’t always supported by popular opinion at the time.
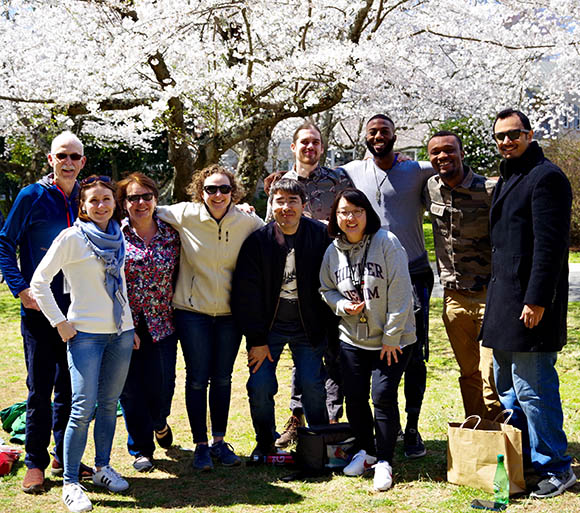
CREDIT: NIDDK
Hanover Lab Picture 2020. Annual trip to the Kenwood Cherry Blossoms. From left to right: John Hanover, Agata Steenackers, Marcy Comly, Lara Abramowitz, Ilhan Akan, Dan Konzman, Hyun-jin Na, Jashua Dawson, Jeffrey Boake, and Mohit Mathew.
What’s next for Hanover? He hopes to move the lab in new directions with his DNA methylation work. “I don’t like to rest on our laurels,” he said, adding that all the new knowledge gained in glycobiology hasn’t yet made it into the general biochemical textbooks—and that there’s much more to be discovered. “I still open the journals and learn something every day, even after studying this for 40 years.”
Read about other NIH glycobiology researchers in “Glycobiology Research at NIH.”
This page was last updated on Tuesday, May 17, 2022