Meet the Makers
NIH Biomedical Engineers Shape Tomorrow’s Technology
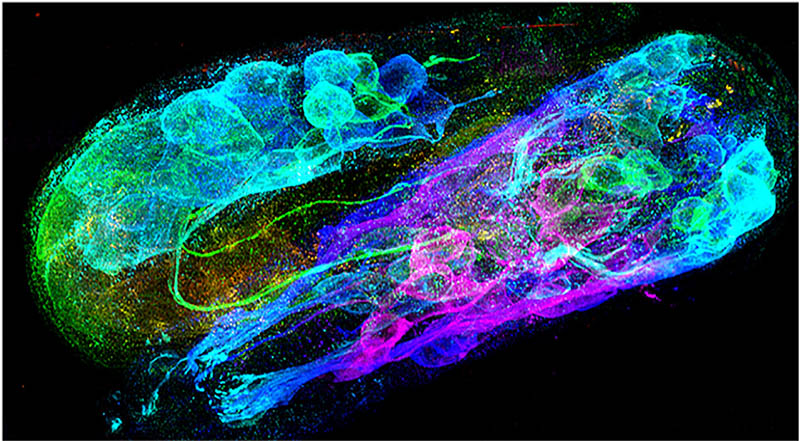
CREDIT: YICONG WU, YIJUN SU, NIBIB
Triple-view scanning confocal super-resolution image of a C. elegans embryo stained with fluorescent antibodies to highlight the neuronal structures.
The unassuming salamander has the remarkable ability to regenerate a lost limb. Could we ever regenerate tissue that way or even grow new body parts? What might hidden biological processes happening at the nanoscale teach us about human health? Can mathematical equations be used to understand and predict the behavior of complex biological systems? How can artificial intelligence be effectively used as a tool for biomedical research?
Bioengineers at the National Institute of Biomedical Imaging and Bioengineering (NIBIB) are asking big questions—and striking up trans-NIH collaborations to answer them. These answers hinge on purpose-driven, practical technologies that are just evolving or have yet to be conceived. From advanced optics and artificial intelligence to new imaging techniques, diagnostics, and biomaterials, NIBIB scientists apply the physical sciences such as physics and chemistry to solve biomedical problems. And by joining forces with researchers across NIH’s intramural research program (IRP), they’re moving discovery into entirely new places.
Team science creates new technology
In December 2019, Kaitlyn Sadtler arrived at NIH and was handed the keys to her lab. As a Stadtman Investigator and head of NIBIB’s Section on Immunoengineering, she was eager to delve into how the body’s immune response might enhance tissue regeneration. But the COVID-19 pandemic in early 2020 soon prompted an abrupt change of course: Her lab quickly refocused, launching a nationwide SARS-CoV-2 survey with the goal of determining the proportion of people with undiagnosed COVID-19 infections to understand how the virus was spreading.
But first, they needed brand new tests: Over 10,000 study volunteers from across the country would eventually mail at-home blood collection kits back to Sadtler’s lab to be tested for the presence of SARS-CoV-2 antibodies, which would indicate a previous infection.
In March 2020, Sadtler’s lab led a cross-institute project to develop enzyme-linked immunosorbent assays (ELISAs) that could detect SARS-CoV-2 antibodies. Dominic Esposito’s Protein Expression Laboratory at the National Cancer Institute (NCI) generated viral proteins that would be essential reagents for the new assays. Broad data-collection efforts were enhanced by capabilities at the National Center for Advancing Translational Sciences. Combined with expertise from researchers at the National Institute of Allergy and Infectious Diseases, a new set of ELISAs were born. Their protocol was published in Nature Communications (Nat Commun 12:article number 113, 2021).
“We now have a panel of 12 ELISAs to look at antibody reactivity against different proteins in the SARS-CoV-2 virus as well as different variants,” said Sadtler.
Finding collaborators
How do investigators with common goals find each other? Often it’s word of mouth, but another way is through scientific interest groups (SIGs). Sadtler helped establish the COVID-19 SIG in 2020 and recently formed the new Biomedical Engineering SIG with fellow bioengineer Matthew Wolf, a Stadtman Investigator at NCI. The latter SIG aims “to integrate the bioengineering community across different ICs,” Sadtler said.
Partnerships outside of NIH—sometimes just across the street—often spark innovation. Sadtler’s immunoengineering lab has resumed its work on regenerative medicine, and one project is with colleagues at Walter Reed National Military Medical Center (Bethesda, Maryland), who are testing a drug to prevent scar tissue from forming in injured rat muscle. The NIBIB team characterizes the immune response to the injury and correlates that with changes in muscle physiology found by the Walter Reed investigators.
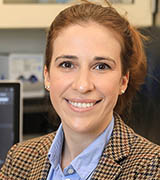
CREDIT: NIBIB
Kaitlyn Sadtler, head NIBIB’s Section on Immunoengineering, explores tissue regeneration.
Further work at Sadtler’s lab focuses on how a natural biologic scaffolding known as decellularized extracellular matrix (dECM) can be used to orchestrate a productive immune response to injury and regenerate tissue. She’s discovered that biomaterials like dECM attract critical immune players such as helper T cells, which then cue stem cells to produce healthy tissue instead of scar tissue. Such technology has shown promise in a variety of clinical settings from wound healing to regenerating damaged muscle and cartilage and might one day be used to regrow entire human organs.
Sadtler has even had conversations with physicians who wonder whether it’s possible to develop ways for surgical wounds to heal faster. “Being a bioengineer is fun,” she said. “We get to bridge that gap between basic studies and talking to a surgeon and asking: ‘What would you actually use if I designed something?’”
AIMing for a closer look
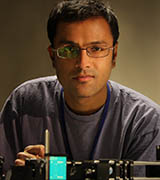
CREDIT: NIBIB
Hari Shroff runs the Advanced Imaging and Microscopy Resource.
NIBIB’s Hari Shroff runs the Advanced Imaging and Microscopy Resource (AIM) in Building 13. A resource for all intramural scientists, this trans-NIH facility offers cutting-edge prototype microscopes paired with high-performance computing that can improve image quality and recording speed. And new systems capable of witnessing the birth of a neuron or marveling at mitochondria in high-resolution are in the works. “I consider myself a tool developer, and I want people to use my tools,” said Shroff, who also leads the Laboratory of High Resolution Optical Imaging (HROI), located just next door to AIM. HROI has been an incubator for instruments now deployed at core facilities throughout campus, including a light sheet microscope and a high-speed super-resolution microscope that were patented by NIH and now produced commercially.
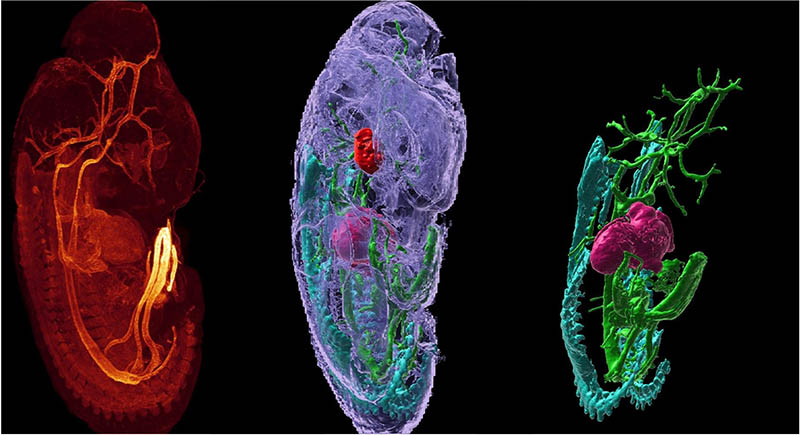
CREDIT: M. LINDHURST, WINCOVITCH (NHGRI); Y. SU, H. VISHWASRAO (NIBIB)
The Advanced Imaging and Microscopy Resource offers cutting-edge prototype microscopes paired with high-performance computing that can improve image quality and recording speed. Shown: A mouse embryo’s fluorescently labeled smooth muscle actin and autofluorescence were imaged using a multi-view light sheet microscope, which rapidly acquires two views of a sample that can be computationally fused into a single high-resolution 3D rendering (left).
Shroff often reaches out to IRP investigators to see what cells and tissues they have that could highlight a new instrument’s capabilities. His group recently published a paper (Nature 600:279–284, 2021) in which they described how their new triple-view line-scanning confocal microscope captured extraordinary super-resolution images. Some of the imaged samples, such as mouse esophagus tissue and proteins interacting within immune cells, were specially prepared by colleagues at NCI and the National Heart, Lung, and Blood Institute (NHLBI).
Robert Fischer at NHLBI studies cell biology in 3D by preparing live cells in collagen gels. He tested HROI’s prototype structured illumination microscope to reveal the inner workings of cells at twice the resolution of conventional microscopes, which is approximately 250 nanometers. The technique rapidly scans a sample, and then machine-learning algorithms fuse together 3D images. While the method is useful for capturing live cellular processes (such as a quickly moving virus), it can distort images in the third dimension, so the HROI team has been improving the optics and algorithms. “Those images now appear [in focus from all directions] with 100-nanometer-scale resolution,” said Shroff. “I think there’s a lot of biology that lives at that scale.”
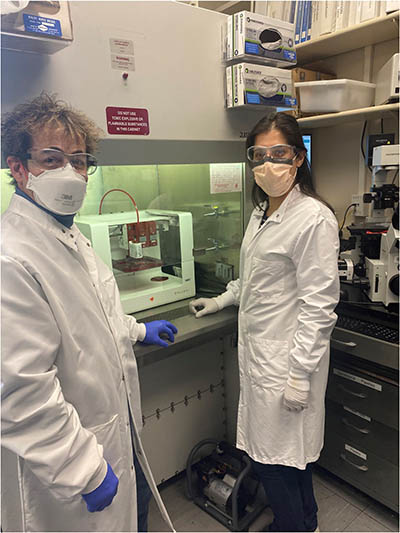
CREDIT: NICOLE MORGAN, NIBIB
Robert Fischer (NHLBI) uses NIBIB’s advanced imaging tools to study the inner workings of cells at ultra-high resolution. Here, Fischer (left) and Paniz Rezvan Sangsari (NIBIB) use an extrusion bioprinter to make artificial vessels.
The bioengineering landscape is rife with instances of ingenuity in which existing technology is modified or used in unexpected ways. Consider adaptive optics: Light distortion entering a lens is measured in real-time and compensated for by a deformable mirror, which corrects the aberration. Astronomers have used the technology for decades in ground-based telescopes to scan the heavens, but in recent years, the technique has been adapted to look within the body. Stadtman Investigator Johnny Tam leads a National Eye Institute (NEI) team that is using a custom-built adaptive optics scanning light ophthalmoscope at the NIH Clinical Center (CC). This instrument enables the team to noninvasively image the retinal cells of people with diseases such as age-related macular degeneration. Back at Shroff’s lab, researchers are integrating adaptive optics into microscopy. They hope it will sharpen blurry images caused by scattered light that occurs over the curved surface of thicker samples.
How to efficiently analyze tomes of data is a challenge that scientists are grappling with. One of Shroff’s prototype light sheet microscopes looks at cleared tissue (tissue made transparent by using solvents). This microscope can collect terabytes of data, say, on an entire mouse brain, in a day. Investigators can then spend months searching for just the key pieces relevant to their work. A biologist sifting through volumes of data on that mouse brain might be tracing a single axon and only be interested in the few instances where it synapses. This is an area that Shroff hopes advances in artificial intelligence can help speed up the search process. “We need biologists to road test our tools currently in development,” said Shroff. They can “highlight the problems…so we know what the next generations of tools should do.”
Meeting technical support needs
Searching for a rheometer on campus? Look no further than the Trans-NIH Shared Resource on Biomedical Engineering and Physical Science (BEPS). Don’t know how to use a rheometer? BEPS can help with that as well. BEPS’s five units can support any NIH scientist in a range of applications whether it’s engineering custom immunoassays and microdevices or providing training on specialized instruments and laboratory techniques.
“We try to be at the leading edge of what people need,” said BEPS Acting Chief Nicole Morgan. She’s currently working with Clare Waterman at NHLBI to understand how forces generated by circulating blood in straight and curved vessels affect the structure of cells lining the vessels’ walls. They’re using a newly purchased extrusion bioprinter to build model blood vessels and are able to quantify the model’s elasticity and stiffness with a rheometer—an important step to ensuring an appropriate environment for cell growth. Understanding such dynamics could yield insights into how immune or cancer cells move throughout the body.
“A lot of the things that we work on are heavily customized and optimized for specific research problems,” said Morgan, who also heads the Microfabrication and Microfluidics Unit. Her team can quickly create multiple versions of a device to arrive at the one that best fits a project.
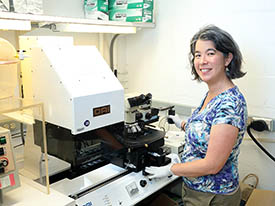
CREDIT: CHIA-CHI “CHARLIE” CHANG, NIH
Nicole Morgan is the acting director for the Trans-NIH Shared Resource on Biomedical Engineering and Physical Science.
Morgan’s lab recently fabricated a device that mimics the branching microvasculature network found in the lung. Researchers at the National Institute of Arthritis and Musculoskeletal and Skin Diseases who investigate autoimmune diseases used it to compare how healthy and diseased neutrophils might navigate through pulmonary capillaries.
Moving to BEPS’s Micro Analytical Immunochemistry Unit, run by Heather Kalish, are the SARS-CoV-2 assays and automated ELISA equipment that have already been used in other IRP projects. Kalish’s team also hosts a new matrix-assisted laser desorption/ionization–time of flight mass spectrometry system, a powerful tool that can be used to generate heat maps of proteins and other organic molecules in tissue sections. The unit also operates the only inductively-coupled-plasma optical emission spectrometry system on campus, which is used to analyze trace metals in biological samples.
BEPS’s three other units—the Electron Microscopy Unit, the Quantitative Methods for Macromolecular Interactions Unit, and the Scanning Probe Microscopy Unit—support a myriad of other IRP projects, too.
Novel instrumentation and design
If you’ve needed assistance testing the auditory function of rodents or endeavored to build a handheld hematoma detector, you may have worked with Tom Pohida’s group of electrical, biomedical, computer, and mechanical engineers. He’s chief of the Instrumentation Development and Engineering Application Solutions (IDEAS) Section, the third and newest trans-NIH shared resource hosted at NIBIB. Formerly under the Center for Information Technology, Pohida’s lab will continue to focus on integrated instrumentation.
“IDEAS cuts across clinical and basic sciences,” said NIBIB Scientific Director Richard Leapman. It “can work with individual PIs in areas including tissue engineering, bioreactors, sensors, electronic components, and 3D-printed clinical devices such as those used in the [NIH Clinical Center].”
Leapman also highlights another of NIBIB’s team science components, the Positron-Emission Tomography Radiochemistry and Imaging Core, led by Dale Kiesewetter. This facility innovates new ways to create biomarkers known as probes, which are used to noninvasively visualize cellular processes in medical diagnostic imaging. In collaboration with other NIH scientists, the group has already developed probes to study biological events from inflammation and cellular metabolism, to cancer metastasis and the growth of new blood vessels.
Improving methodology
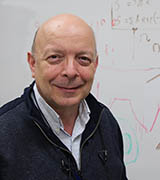
CREDIT: RAYMOND MACDOUGALL, NIBIB
Carlo Pierpaoli, who is head of NIBIB’s Laboratory on Quantitative Medical Imaging, is a pioneer in the field of diffusion magnetic resonance imaging.
“We are not just providing technical support, we’re working in partnership with [clinicians] to develop new methods,” said Carlo Pierpaoli, head of NIBIB’s Laboratory on Quantitative Medical Imaging (QMI). Pierpaoli is a pioneer in the field of diffusion magnetic resonance imaging (dMRI), which allows researchers to visualize the size and microstructure of white matter in the brain. The technique is being used to gain insight into healthy brains as well as the brains of people who’ve had a stroke or who have neurodegenerative disorders such as Alzheimer disease. (Magn Reson Med 85:2696–2708, 2021). As they continue to refine their craft, the QMI lab created a software suite called TORTOISE that makes their methodologic improvements available to any researcher and is currently being used at other neuroscience centers to analyze dMRI data.
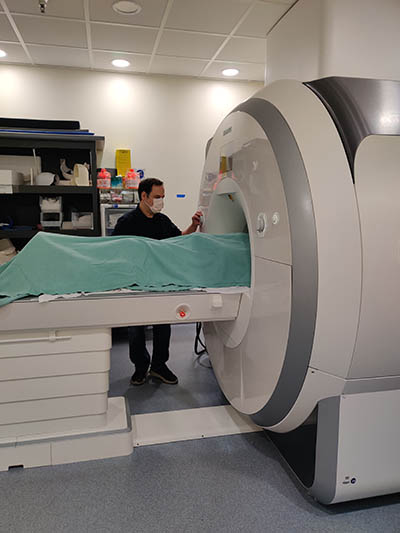
CREDIT: CARLO PIERPAOLI, NIBIB
Staff scientist Okan Irfanoglu in NIBIB’s Laboratory on Quantitative Medical Imaging, is performing an MRI scan of a research subject.
Pierpaoli is also working with a European group that uses focused ultrasound to treat essential tremor, a debilitating condition resulting in uncontrolled limb movement, making basic tasks such as eating nearly impossible. The FDA-approved treatment uses ultrasound to heat and destroy the small patch of brain tissue responsible for the tremor. But the surgical planning is imprecise. Clinicians currently use anatomical landmarks and lower-intensity ultrasound to localize where the final treatment should be, which could accidentally damage some healthy brain tissue. The dMRI methods could minimize that risk by creating an accurate map of the brain to more precisely determine where the ultrasound should be directed. Pierpaoli has already seen his technology used successfully.
He advocates for getting out of the lab to see what questions others are asking. He has lectured at international clinical conferences and presented at the CC’s grand rounds. “You need to stick your neck out of your own lab and listen to the clinicians, it is important to become aware of unmet needs that you may be able to address,” he said.
Accelerating bioengineering at NIH
A thread of bioengineering weaves throughout NIH. “There’s just not enough of it,” said NIBIB Director Bruce Tromberg. “But the demand is extremely high.”
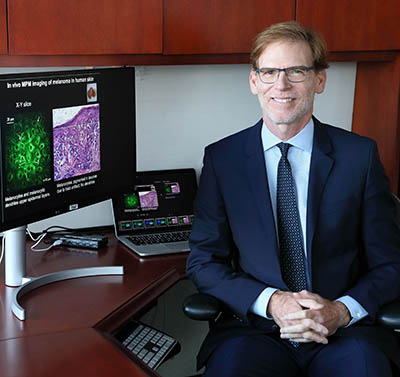
CREDIT: CHIA-CHI “CHARLIE” CHANG, NIH
NIBIB Director Bruce Tromberg is planning an NIH Center for Biomedical Engineering and Technology Acceleration, which will unite multidisciplinary teams of technology developers on campus and facilitate the enhancement of synthetic biology and new biomaterials, artificial intelligence, and wearable sensors paired with digital health platforms that help patients self-manage their conditions.
Enter the NIH Center for Biomedical Engineering and Technology Acceleration (BETA), Tromberg’s bold vision for a centralized entity that will unite multidisciplinary teams of technology developers on campus. BETA will provide the people, culture, and tools to propel technology-driven interdisciplinary research, training opportunities, and clinical translation at NIH.
The new center will draw from existing NIBIB core facilities and resources. Spaces in Building 13, the Porter Neuroscience Research Center (Building 35), and the CC have been identified as places that will accommodate a growing team of innovators and state-of-the-art equipment. As it evolves, Tromberg hopes that BETA will facilitate joint appointments within the IRP, attract biomedical engineers from outside of NIH, and have the potential to form industry partnerships and commercialize new technology. BETA could facilitate the enhancement of synthetic biology and new biomaterials, artificial intelligence, and wearable sensors paired with digital health platforms that help patients self-manage their conditions. The groundwork for research programs is being laid now and a BETA director is expected to be appointed by this summer.
Tromberg points to the enormous growth of the country’s more than 140 biomedical engineering departments—many combining engineering and medical schools—that have become centers of innovation and retain energy and talent through an entrepreneurial startup ecosystem. He envisions the same happening at NIH. “That would be a great outcome, and a logical one.”
Bioengineers are a practical bunch, united in their ambition to bring new technologies from an idea to a tool that contributes meaningfully to human health. And according to Tromberg, the possibilities are limitless. “Technology itself is never done,” he said. “There are always ways to continue to push the envelope.”
To learn more about how NIBIB can help you with your projects, go to https://www.nibib.nih.gov/labs-at-nibib.
To collaborate with NIBIB’s shared resources, email them directly at:
- AIM: hari.shroff@nih.gov
- BEPS: morgann@mail.nih.gov
- IDEAS: IDEAS-info@mail.nih.gov
This page was last updated on Tuesday, May 17, 2022