From Deep Sea Vents to Our Own Stomachs
Demystifying How Bacteria Thrive in Extreme Environments
What could microorganisms that exist in extreme environments such as hot springs in the crushing depths of our oceans have to do with bacteria that reside in our own bodies? This is the question that the “Demystifying Medicine” lecture series attempted to answer on February 5, 2019. Bridging a huge gap, from 2,500 meters under the sea to inside our own intestines, Stefan Sievert and John Dekker (National Institute of Allergy and Infectious Diseases, NIAID) explained how intricately connected these two worlds are and how new genomic technologies can help us understand the evolution of these microorganisms.
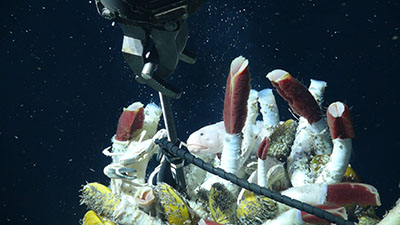
CREDIT: PHOTO COURTESY OF STEFAN SIEVERT, WHOI/NSF/ROV JASON, © WOODS HOLE OCEANOGRAPHIC INSTITUTION
The manipulator arm on the remotely operated, deep-sea vehicle Jason uses a sensor to measure the oxygen concentration of warm hydrothermal fluids discharging from a hydrothermal vent surrounded by a community of tubeworms and mussels at a site called “Crab Spa” at a depth of 2,500 meters at the deep-sea hydrothermal vent field at 9ºN on the East Pacific Rise. Hanging out is also a zoarcid fish and vent crab.
Sievert is a microbial ecologist at the Woods Hole Oceanographic Institution in Falmouth, Massachusetts. His primary interest is the microbes that live in deep-sea hot springs or hydrothermal vents. These vents form along mid-ocean ridges, where new ocean crust is formed by sea-floor spreading as magma rises to the sea floor at the rifts of tectonic plates.
At these undersea volcanoes, cold deep-sea water that enters the ocean crust is heated due to an underlying magma chamber. During this process, the seawater reacts with rocks at high temperature and pressure, transforming them into a so-called hydrothermal fluid that rises back to the sea floor. Where the torching hot, anaerobic vent fluid exits the seafloor and comes into contact with the cold, oxygenated deep-sea water, minerals precipitate, creating chimneylike structures and distinctive “black smoke.”
Temperatures around the vents vary from 662 degrees Fahrenheit at the black smokers to 41 degrees Fahrenheit where the vent fluid mixes with the ambient deep-sea water, either below or above the sea floor. The warm temperature gradients coupled with chemical compounds from the vent fluids create a chance for life to thrive even in the dark and food-starved deep sea.
Instead of getting energy from light, as plants do in photosynthesis, these microbes use chemical energy in a process called chemosynthesis. The microbes form the base of the food chain at these highly productive ecosystems that were only discovered about 40 years ago. The microbes use chemical compounds such as hydrogen sulfide and hydrogen rising out of the vents, along with oxygen and nitrate from the deep-ocean water, to create the biological compounds needed for life from carbon dioxide. By forming symbioses with these chemosynthetic bacteria, other organisms such as tube worms can live along the vents as well.
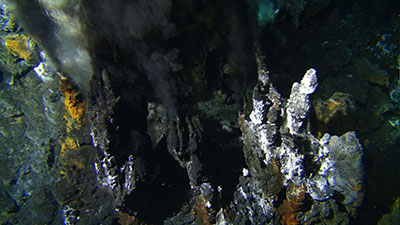
CREDIT: PHOTO COURTESY OF STEFAN SIEVERT, WHOI/NSF/HOV ALVIN, © WOODS HOLE OCEANOGRAPHIC INSTITUTION
Microorganisms that exist in deep-sea hydrothermal vents like this one have a lot in common with the microorganisms that live inside our bodies. Relatives of Campylobacter (which causes food poisoning), Helicobacter (which causes ulcers), and Arcobacter (a Campylobacter-like organism that can tolerate oxygen) are all found along the deep-sea vents.
As Sievert showed images of deep-sea vents and otherworldly creatures drifting by a deep-ocean submersible vehicle, it was hard to imagine the connection to human health. Yet many of these bacteria are closely related to those that live, and cause disease, in our own bodies. Relatives of Campylobacter (which causes food poisoning), Helicobacter (which causes ulcers), and Arcobacter (a Campylobacter-like organism that can tolerate oxygen) are all found along the deep-sea vents.
While not genetically identical to their land-locked relatives, these bacteria do have some similar traits including a glycosylation pathway that aids in interactions with any host species. The bacteria also tend to be highly adaptive, for example by having highly plastic genomes due to a lack of DNA-repair mechanisms, perhaps giving them the unique ability to exist in extreme environments such as the deep oceans and the human intestinal system.
Although the research in Sievert’s lab mostly focuses on understanding the ecological and biogeochemical roles of these microbes, an important question is, “How did these bacteria come to live inside a host species on the Earth’s surface?” Did the sea and terrestrial bacteria evolve together as the first creatures were transitioning from aquatic to terrestrial life, or did the evolution take place later? Although these questions are still unanswered, new genomic techniques introduced by the next speaker, John Dekker, may hold the secret to unlocking this mystery.
Dekker, a Lasker Clinical Research Scholar and chief of the Bacterial Pathogenesis and Antimicrobial Resistance Unit in NIAID, is also the director of the Genomics section of NIH Clinical Center’s Microbiology Service. Although he did not talk about the same bacteria as Sievert, Dekker reviewed recent advances from labs around the world involving the application of genomic techniques to identify other human pathogens—such as Leptospira (a bacterial infection that is passed from animals to humans), astrovirus (a major cause of acute diarrhea in children and a rare cause of encephalitis), and Ebola virus.
The techniques Dekker reviewed start with taking specimens from an infected patient and applying high-throughput sequencing to generate a huge number of short “reads” of the DNA base-pair sequences, which are then analyzed computationally. All the human reads are filtered out, and the remaining reads are cross-referenced against databases of bacterial, viral, and fungal pathogens.
These broad-scale analyses allow the identification of new and rare organisms that traditional methods might not otherwise detect. These techniques have been used to diagnose rare diseases, such as infection with Leptospira, which can cause fever and infection of the brain, and to track the evolution of Ebola outbreaks in West Africa. The quick and accurate diagnosis of pathogens allows for targeted treatment and for improved containment protocols to be put in place.
An even newer technology, nanopore sequencing with a pocket-sized sequencing device, allows this analysis to be completed faster and with greater accuracy. During the 2015 Ebola outbreak, on-site use of nanopore sequencing allowed researchers to track the evolution of the virus in real time. Dekker also presented work from his own lab in which it used rapid nanopore sequencing to identify antibiotic resistance mechanisms in bacteria.
The application of genomic techniques helps researchers understand how bacteria evolved to develop resistance to antibiotics and adapt to new environments. Perhaps these same techniques applied to disease outbreaks can answer the question of how deep-sea bacteria came to play a part in our day-to-day lives.
To see a videocast of the whole lecture, go to https://videocast.nih.gov/launch.asp?27302. In addition, both speakers selected additional reading for interested audience members—they can be found at https://demystifyingmedicine.od.nih.gov/DM19/CourseMaterials19.html. To read an NIAID blog post on the event, go to https://www.niaid.nih.gov/news-events/under-sea-and-your-guts. And to find out more about the DeMystifying Medicine series, go to https://demystifyingmedicine.od.nih.gov.
This page was last updated on Monday, April 4, 2022