The SIG Beat
News From and About the NIH Scientific Interest Groups
Proteostasis
One Name, Many Fields: “Proteostasis” Research at NIH
Proteostasis, a seemingly straightforward fusion of the words “protein” and “homeostasis,” is actually a fertile and multifaceted concept. Ask any 10 scientists to define it, and you’re bound to get 10 different answers, and those opinions may change from year to year.
In effect, proteostasis is greater than the sum of its parts, encompassing the study of all areas of protein health and fitness that contribute to maintaining cellular integrity and function. Derailed proteostasis leads to protein misfolding and aggregation that is known or suggested to be implicated in many devastating human disorders including neurodegenerative diseases such as Alzheimer disease, Parkinsonism, amyotrophic lateral sclerosis, type 2 diabetes, cancer, cardiomyopathy, cystic fibrosis, cataracts, prion disease, immune problems, metabolic deficiencies, alcoholic liver disease, and other chronic maladies. It is well established that proteostasis naturally declines during aging.
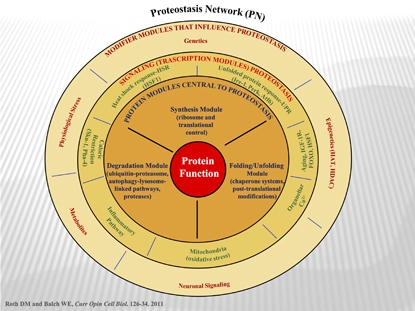
A. Orosz, NIAAA
The Proteostasis Network (PN): Wheel of fortune. A simplified (or more complicated?) overview of the proteostatis concept. The PN supports protein function by generating and maintaining the biological protein fold. The innermost layer represents the General Proteostasis Control triad and includes the synthesis module, the folding/unfolding module, and the degradation module commonly. The second layer shows the signaling transcriptional pathways that influence the level and activity of the triad. The outer layer includes modifiers that influence and/or integrate the activities defined by the second and first layers. Modifiers and signaling pathways shown are from both cell autonomous and cell non-autonomous origins.
At the NIH, the processes underlying such conditions traditionally have been studied in isolation, with fields of study delineated by disease classifications. But Andras Orosz—a program director at the National Institute of Alcohol Abuse and Alcoholism and driving force behind the recent formation of the Proteostasis Scientific Interest Group (SIG)—thinks that it’s time we change our approach to understanding and treating these devastating chronic diseases.
He believes that “learning the basic biology of proteostasis is important for understanding a slew of debilitating protein misfolding and aggregation disorders and [for] developing cures.”
As such, the Proteostasis SIG brings together intramural research program (IRP) investigators from across multiple fields and institutes to share knowledge about the underlying molecular commonalities of different diseases.
Proteostasis, a term coined in a landmark Science paper in 2008, is a broad concept that is relevant to all fields of study that involve protein synthesis, folding, processing, and turnover (Science 319:916–919, 2008). Some major subgroups that fall under the proteostasis umbrella include protein synthesis and degradation systems; chaperone proteins that bind to nascent polypeptide chains and partially folded proteins to ensure proteins attain correct conformation; and post-translational modifications that can modulate protein activation states, specify intracellular location, or tag a protein for degradation by the proteasome (a specialized organelle that breaks down proteins and recycles their amino acids). As many as 2,000 proteins are involved in the proteostasis network.
The decline of proteostasis with aging
When protein homeostasis degenerates and collapses, cells get old . . . and sick. Hallmark features of cellular aging include a buildup of proteotoxic stress, mistranslation of nascent polypeptides, and concentration of damaged, misfolded proteins and protein aggregates. This accumulation of cellular waste is toxic to cells. That’s when things start to fall apart.
Senior Investigator Jay Chung, head of the National Heart, Lung, and Blood Institute’s (NHLBI) Laboratory of Obesity and Aging Research, believes that the same molecular pathways that are affected under conditions of metabolic stress go awry in natural aging. The common thread between these seemingly disparate disorders boils down to the issue of unbalanced proteostasis. Chung says that understanding how these stresses affect protein quality control is tantamount to understanding how and why aging-associated diseases such as Alzheimer disease and obesity-related health pathologies develop.
Central to quality control of proteins in the cell is the molecular chaperone heat shock protein 90 (HSP90). Chung has observed that post-translational modifications of HSP90 can be drastically altered in either aged or obese mice compared with healthy and younger animals. He has also demonstrated that calorie restriction, a method previously shown to increase longevity in mice, can reverse the post-translation modifications associated with aging and obesity, restoring proteostasis on a molecular level. The full spectrum of mechanisms by which age and caloric restriction affects proteostasis are currently unknown and under intense investigation.
Elsewhere in the NHLBI, Toren Finkel and his team in the Laboratory of Molecular Biology are interested in understanding the basic mechanisms underlying natural aging, with the idea that if one can slow the aging process, it may be possible to alter the rate at which a variety of these age-associated diseases occur.
His lab recently showed that lowering the expression levels of a gene encoding a signaling molecule called mammalian target of rapamycin (mTOR) can increase the lifespan of mice by up to 20 percent. This feat would be equivalent to raising the average lifespan of a human being from 75 to 90 years (Cell Rep 4:913-920, 2013).
Finkel says that one potential mechanism by which mTOR controls life expectancy is through the proteostasis degradation pathway of autophagy. Autophagy, a process by which cells clear damaged organelles and proteins, is essential to maintaining healthy protein homeostasis.
“The trash can build up either if you make more trash or [if] you don’t throw it away,” Finkel said. “Autophagy is how you throw it away. If that gets screwed up, then that could contribute to some of the issues of aging that we’re interested in.”
Protein aggregates, amyloid, and prions
Protein aggregates are one type of cellular trash that can accumulate when the proteostatic balance is lost.
Amyloids are a form of protein aggregate comprising filaments of monomers bound by hydrogen bonds. Formation of amyloid is pathogenic in several neurological disorders including Huntington, Lou Gehrig’s, and Alzheimer diseases. Other neurodegenerative diseases, such as Creutzfeldt-Jakob disease and bovine spongiform encephalopathy, are caused by pathogenic amyloid structures comprising misfolded prion proteins.
While it is known that these disorders arise through disparate genetic mechanisms and that the aggregates are formed from different proteins, there are striking similarities in their three-dimensional structures. Understanding the basics of how and why these proteins aggregate may provide clues about how to break apart amyloid plaques and potentially reverse disease pathologies.
Reed Wickner, chief of the Laboratory of Biochemistry and Genetics in the National Institute of Diabetes and Digestive and Kidney Diseases (NIDDK), uses yeast prions as a model to study the dynamics of amyloid structures. One finding from his group that may yield clues about amyloid turnover is the identification of a protein, Batten-disease-related protein 2 (Btn2), that cures yeast cells of prions by sequestering prion aggregates to a cellular compartment.
Some 2,300 miles away from Bethesda, at the Rocky Mountain Laboratories (Hamilton, Mont.), which is part of the National Institute of Allergy and Infectious Diseases (NIAID), researchers are seeking to understand prion biology in mammalian systems. Suzette Priola, chief of the Transmissible Spongiform Encephalopathy (TSE)/Prion Molecular Biology Section, has identified a region of mammalian prion protein that controls interspecies transmission. Her group is also making progress in deciphering the early stages of the disease, in particular, those events that trigger initial prion protein misfolding.
Molecular chaperones
Molecular chaperones are critical components of the proteostasis network. These cellular guardians are ubiquitous in cells and yet with the assistance of their co-chaperones maintain high specificity for their client proteins. Chaperones also bind to newly synthesized polypeptide chains to ensure proper folding and prevent protein aggregate accumulation.
Senior Investigator Daniel Masison, in NIDDK’s Laboratory of Biochemistry and Genetics, is exploring mechanisms by which chaperones target specific prion aggregates. He has shown that heat shock protein 70 (HSP70) acts with co-chaperones to propagate prion replication by breaking up amyloid structures into fragments, each of which can then seed a new prion aggregate.
Masison collaborated with NHLBI’s Lois Greene to demonstrate that overexpression of co-chaperone HSP104 resulted in complete disintegration of prion aggregates in hours.
Greene’s laboratory is using real-time cell imaging to study mammalian prion biology as well as the function of chaperone proteins in the formation of clathrin-coated pits, a mechanism by which cells can internalize cargo-bound receptors on the cell surface.
Lisa Cunningham, head of the Section on Sensory Cell Biology in the National Institute on Deafness and Other Communications Disorders, is investigating a lesser-known role for the molecular chaperone HSP70. She and her team have discovered that HSP70 secreted by neighboring cells is a critical factor in protecting mechanosensory hair cells in ototoxic-mediated hearing loss. Traditionally the roles of chaperones and heat-shock proteins in protein folding and trafficking were thought to be limited to folding and stabilizing proteins within the cell in which they are synthesized.
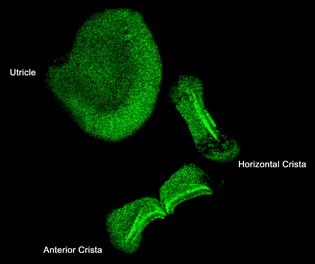
L. Cunningham, NIDCD
Lisa Cunningham (NIDCD) discovered that heat shock protein 70 (HSP70) protects mechanosensory hair cells from the ototoxic side effects of certain therapeutic drugs. She is working with the NIH Clinical Center to restore hearing loss by inducing HSP70 expression in patients’ inner ears. Shown here: HSP70 expression in supporting cells in the vestibular organs of the mouse inner ear.
Cunningham is working with the audiology team at the NIH Clinical Center to restore hearing in patients by inducing HSP70 expression in the inner ears.
Proteostasis and cancer
Leonard Neckers and his group at the National Cancer Institute’s (NCI) Urologic Oncology Lab, like Chung, also are interested in the chaperone protein HSP90. Neckers has found that HSP90 is a critical factor for cell survival and proliferation in many types of cancers. Called the “cancer chaperone,” HSP90 works to stabilize proteins in the cell, many of them protein kinases, that play important roles in cell growth, cell survival, apoptosis, and oncogenesis.
But stabilization is not always a desirable outcome. In certain types of cancers, HSP90 stabilizes oncogenic proteins, shielding them from degradation. Thus, overactive HSP90 can sustain cancer-cell growth and survival by extending the lifespan of toxic proteins. In the late 1990s, Neckers’s lab identified the first small-molecule HSP90 inhibitor and helped develop the first-in-human drug targeting HSP90. Several chemically distinct HSP90 inhibitors are currently in phase 1 and phase 2 clinical trials and one is in phase 3 clinical trial.
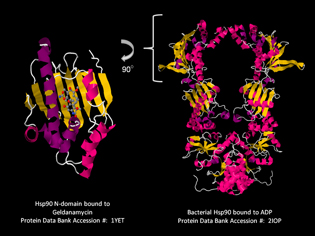
L. Neckers, NCI
Len Neckers (NCI) has found that the chaperone protein HSP90, which stabilizes proteins in the cell, is a critical factor for cell survival and proliferation in many types of cancer. His lab identified the first small-molecule HSP90 inhibitor and helped develop the first-in-human drug targeting HSP90. Pictured: crystal structures of HSP90.
Allan Weissman, chief of NCI’s Laboratory of Protein Dynamics and Signaling (Frederick, Md.), has focused his efforts on understanding the ubiquitin system, which he describes as “the major player in regulating levels of cellular proteins.” Ubiquitination is a post-translational modification that can target proteins for proteasomal degradation. In 1999 Weissman and co-workers were the first to describe (RING)-finger proteins as ubiquitin ligases. RING fingers are a family of enzymes that tag proteins for degradation and constitute the majority of ubiquitin ligases. Although all the targets of RING-ubiquitin ligases have not yet been identified, Weissman has shown that overexpression of a specific RING-ubiquitin ligase leads to a marked enhancement of metastasis—the primary cause of death in cancer.
Proteostasis research at the NIH is far greater than what this article could summarize. As evidence of this emerging field’s importance within the IRP, it made a splash with a well-attended workshop in June and symposium in September and was featured at the NIH Research Festival in November 2013. To learn more about the Proteostasis SIG and related activities, contact Andras Orosz at orosza@mail.nih.gov.
This page was last updated on Thursday, April 28, 2022