New Methods
New Microscopes That Reveal Cellular Processes in 3-D
May Help Scientists Observe How Viruses Attack
NIH researchers have developed two new high-resolution microscopes, both the first of their kind. The first—an instant linear structured illumination microscope (iSIM)—captures high-resolution images of small, fast-moving cellular structures in real time. The second—a dual-view inverted selective plane illumination microscope (diSPIM)—displays large cell samples in three dimensions (3-D) while decreasing the amount of harmful light that cells are exposed to during imaging.
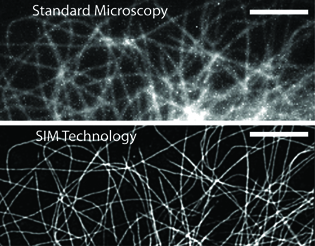
NIBIB
The resolution differences between standard microscopy (top) and SIM technology (bottom) can be clearly seen.
Instant super resolution imaging
“Much of biology depends on the movement of very small proteins finding each other and interacting,” said Hari Shroff, chief of the High Resolution Optical Imaging Lab in the National Institute of Biomedical Imaging and Bioengineering (NIBIB). “We really need to look at how things move in a live cell.”
But it’s difficult to obtain good quality, high-resolution images in a living, moving cell. Traditional linear structured illumination microscopy (SIM), which can provide high resolution in a fixed cell, stumbles when it comes to imaging elements moving quickly in a live cell. The higher the resolution, the harder it is to eliminate the blur that comes from light diffraction and motion.
Shroff and research fellow Andrew York (NIBIB), along with other NIH scientists, developed the iSIM to address these problems. Building on traditional SIM technology, the iSIM allows real-time, 3-D super resolution imaging of live cellular processes such as blood cells racing through a zebrafish embryo. This kind of imaging is impossible with other microscopes (Nature Meth 10:1122–1166, 2013).
Most high-resolution microscopes, including SIMs, use imaging software to capture, store, and combine multiple camera exposures into crisp, exquisitely detailed images. Shroff and his lab equipped an iSIM microscope with powerful lenses and mirrors to produce high-resolution images from the original exposures more quickly.
“Before, we relied on computer software and algorithms to do things like sort through hundreds of images, eliminate out-of-focus light, and combine the individual images together,” said Shroff. The process was time consuming and could take hours or even days. “Now, we can do most of that optically with the microscope itself.” In addition, the iSIM data use only one percent of the hard-drive space that other types of high-resolution microscopes do.
The diSPIM
The second microscope builds on technology called selective plane illumination microscopy (SPIM) that uses a thin beam of light to illuminate only a single plane of tissue at a time. (Traditional microscopes expose a whole sample to light that can damage or even kill biological samples.) The SPIM then rotates the sample so it can be imaged from many angles to create a 3-D effect. But the rotation process slows the imaging speed, making it difficult to capture live cellular motion. Ironically, the sample is still at risk of light damage because it is repeatedly illuminated as each plane is imaged.
To fix this problem, Shroff, NIBIB staff scientist Yicong Wu, and colleagues from NIH and other institutions developed the dual-view inverted SPIM (Nature Biotechnol DOI:10.1038/nbt.2713). The diSPIM can image cellular processes at high speed without causing extensive light damage. Two detection cameras, set at a 90-degree angle to each other, capture perpendicular views of each sample. With relatively simple modifications, traditional single-camera SPIMs can be converted into the dual-camera diSPIM. But to combine the images from the two cameras, the scientists had to create a new post-processing software algorithm.
With the diSPIM microscope, scientists can obtain high-resolution images of live cellular processes—such as fast-moving viruses, migrating cancer cells, and interacting neurons—in a 3-D environment.
“As scientists, it’s up to us to find ways to observe cells as accurately as possible,” Shroff said. “There are a lot of biological processes that we can’t understand without observing them and that’s something these devices can help us do.”
This page was last updated on Thursday, April 28, 2022