Slaying Sickle Cell
Foundational Work at NIH and Today’s Transformative Treatments
BY MICHAEL TABASKO, THE NIH CATALYST
In 1958, biochemist Makio Murayama was recruited to the NIH to study sickle cell disease (SCD), then known as sickle cell anemia. Famously, he constructed a meticulous three-foot-tall 3D model of a hemoglobin protein in his home basement laboratory to work out the molecular mechanisms behind why red blood cells sickle. The misshaped cells are a hallmark of the disease that can occlude circulation, leading to organ damage, stroke, anemia, and sudden episodes of pain.
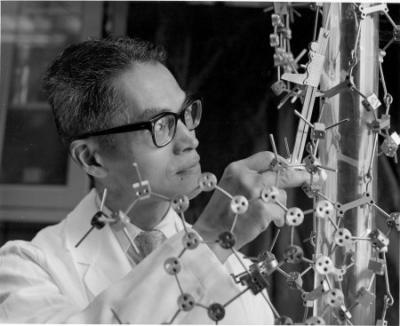
CREDIT: JERRY HECHT, OFFICE OF NIH HISTORY AND STETTEN MUSEUM
NIH scientist Makio Murayama with the molecular model of hemoglobin that he built to study SCD and explain why red blood cells take on a sickle shape.
In his NIH oral history, Murayama expressed his penchant for working alone, his solitary endeavors perhaps analogous to the paucity of research devoted to understanding SCD at that time. Down the road, the work would ultimately pay dividends, and his conclusions about hemoglobin dynamics would inform the first drugs used to treat the disease (PMID: 4952917).
Science has since steamed ahead, and for the 100,000 people in the United States and 8 million people worldwide with SCD, there are now two big reasons to be optimistic.
Two FDA-approved gene therapies for SCD have been added to the armament of potentially curative therapies, which until recently was solely the realm of stem cell transplants requiring a genetically matched donor’s healthy tissue. While tantalizing, these transformative new genetic approaches cost in the millions of dollars, are risky and cumbersome to administer, and don’t work for everyone. But they do represent a future in which a cure is available to more people.
Formative years
The discovery in several laboratories that sickle hemoglobin formed long fibers inside red blood cells, which impaired circulation and drove disease manifestations, renewed interest among a handful of NIH investigators in biophysical studies that would eventually lead to treatments.
“When I came to NIH in 1965, SCD was presented as an example of the accomplishments of modern biochemistry in determining that the abnormality in hemoglobin was due to a single mutated gene,” said Christian B. Anfinsen Distinguished Scientist Alan Schechter, senior investigator at NIDDK’s Molecular Biology and Genetics Section, Molecular Medicine Branch.
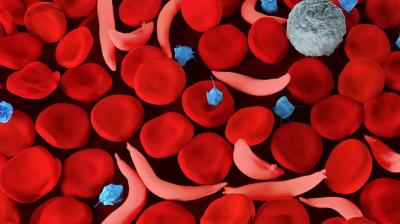
CREDIT: NHLBI
Illustration of sickle red blood cells and normal red blood cells. The misshapen cells occlude circulation and can result in disease manifestations including sudden pain crises, chronic pain, organ damage, and anemia.
Approved drugs were still decades away. Schechter and colleagues focused on using new methods at that time, such as nuclear magnetic resonance, to understand the thermodynamics of how sickle hemoglobin molecules aggregate or polymerize inside deoxygenated red blood cells.
Other foundational scientists, including William Eaton, chief of NIDDK’s Biophysical Chemistry Section, Laboratory of Chemical Physics, elucidated both the thermodynamics and kinetics of sickle hemoglobin fiber formation (PMID: 4531026) and showed how diluting the concentration of sickle hemoglobin could prevent sickling (PMID: 3603036). After more than 50 years of research on SCD, Eaton is still at it today and focused on drug development. In collaboration with several NHLBI teams, he developed a quantitative screening assay and identified more than 100 antisickling compounds, 20 of which could be drug candidates (PMID: 36161945).
Also, genomic work at NIH in the 1970s aimed at one day developing a gene therapy. Scientists including Phillip Leder, Gary Felsenfeld, and others explored the structure of the hemoglobin genes and how they were transcribed into sickled hemoglobin, normal hemoglobin, and fetal hemoglobin.
Fetal hemoglobin, which typically declines after birth, became the prime therapeutic target because it slows the kinetics of the polymerization of sickle hemoglobin and had been associated with less severe forms of SCD. “Back then there were discussions of genetic ways to increase fetal hemoglobin, and we were doing biophysical studies to determine how much was necessary to get effective therapy for SCD,” said Schechter (PMID: 2447498) .
Ensuing laboratory and clinical work at the NIH and elsewhere led to extensive interest in hydroxyurea (HU) as an agent of choice for increasing fetal hemoglobin with minimal side effects. More support for the potential use of HU came from a detailed study of 10 patients at the CC (PMID: 1690857) under the direction of Griffin Rodgers, now NIDDK director.
That study’s success led to a multicenter study of HU in almost three hundred sickle cell patients, supported by NHLBI, which provided definitive evidence that the drug reduced painful crises and blood transfusions (PMID: 7715639). In 1998, HU became the first FDA-approved drug for treating adults with SCD, and in 2017 the FDA approval was extended to pediatric patients over two years of age. According to Rodgers, while not curative, it gave people the opportunity to pursue and enjoy their lives more fully than before.
“I am proud to say that so much of our understanding of SCD, from how it is inherited to the amino acid change responsible for the creation of sickle-shaped blood cells, has been uncovered through research funded by or conducted at the NIH,” Rodgers told the Catalyst, adding that, while promising, current treatments do not work for every patient with SCD, and access to life-changing therapies can be limited due to where they live and other social and economic factors. “Addressing health disparities will be necessary for the development of treatments that are effective for even more people living with SCD and that are accessible to all who need them.”
From new drugs to genetic cures
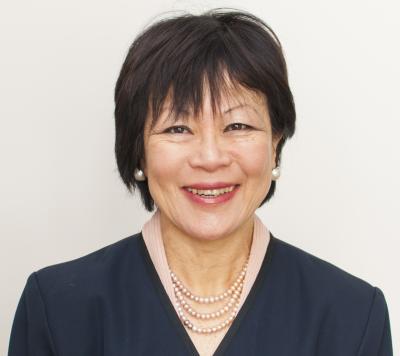
CREDIT: NHLBI
Recent Shaw Awardee Swee Lay Thein’s discovery that the BCL11A gene was involved in turning fetal hemoglobin off at birth led to using a CRISPR-based gene editing approach to disrupt that gene’s function and resulted in one of the newly approved gene therapies for SCD and beta-thalassemia.
Boosting fetal hemoglobin is also behind how one (exagamglogene autotemcel) of the new gene therapies works, but unlike HU, it does so permanently. When NHLBI’s Swee Lay Thein was at Kings College (London), she conducted a genome-wide association study (GWAS) and discovered that the BCL11A gene suppressed fetal hemoglobin at birth, a finding for which she was awarded the prestigious 2024 Shaw Prize in Life Science and Medicine. The discovery made possible the current CRISPR-based gene editing approach that disrupts the BCL11A gene’s function and turns back on fetal hemoglobin. According to the FDA, the treatment was effective in a remarkable 93.5% of patients with SCD and was soon after approved for treatment of beta-thalassemia, a related disorder.
Other scientists, such as Ambroise Wonkam at Johns Hopkins University (Baltimore), aren’t stopping at the BCL11A gene. Wonkam is running GWASs on people of African ancestry to discover even more gene therapy targets that affect fetal hemoglobin (PMID: 36815490).
Thein, who is now chief of the Laboratory of Sickle Cell Genetics and Pathophysiology, is running a clinical trial of the drug mitapivat as another way to mitigate the sickling process, the root cause of the disease. “My focus and research interests are to develop more small-molecule drugs that can reach far, far more patients,” she told the Catalyst.
Listen to Ambroise Wonkam give a 2022 WALS talk and a 2024 Demystifying Medicine talk.
Learn more about Swee Lay Thein’s discoveries in this Research in Action article.
The second approved gene therapy (lovotibeglogene autotemcel) also has an NIH story. “When I got here in the 1990s, I was interested in developing gene therapy using a patient’s own bone marrow cells modified by a viral vector to modify the disease,” said John Tisdale, senior investigator and chief of NHLBI’s Cellular and Molecular Therapeutics, who was instrumental in starting the NIH stem cell transplant program for SCD. “We knew bone marrow transplant worked because it worked for a patient with leukemia who also had SCD and she was cured of both.”
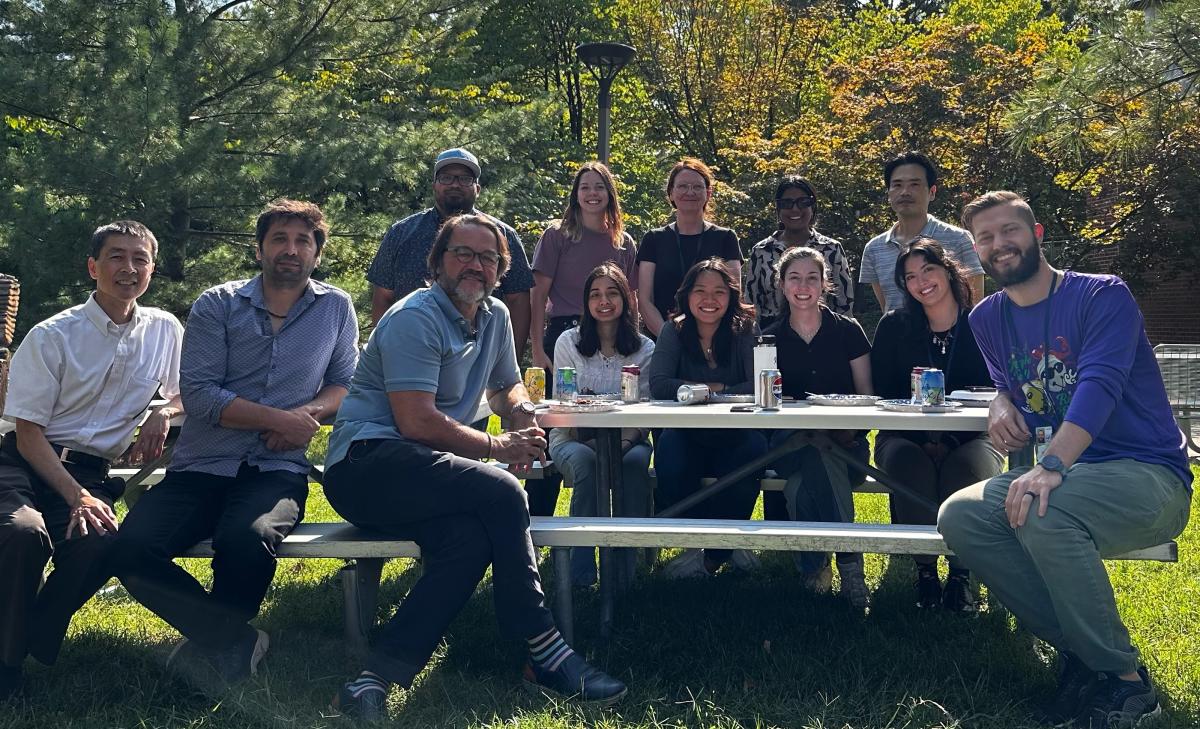
CREDIT: BJORG GUDMUNDSDOTTIR
The 2024 Cellular and Molecular Therapeutics Laboratory staff, led by John Tisdale. Front row (from left to right): Matthew Hsieh, Selami Demirci, John Tisdale, Josiah Ballantine. Middle row: Shruti Sathish, Anh Le, Avery Bradley, Melody Engle. Back row: Oswald Phang, Julia Ball, Bjorg Gudmundsdottir, Henna Butt, Naoya Uchida.
Tisdale developed the first stem cell transplant program for SCD at NIH decades ago, and his team was instrumental in developing lovotibeglogene autotemcel, one of two newly approved gene therapies. Ongoing efforts at his lab are addressing cost, delivery, and efficiency hurdles to one day make gene therapies accessible to more people across the globe.
Tisdale’s team, including Matthew Hsieh, senior staff clinician at the CC who has worked with scores of patients with SCD, began optimizing allogenic, or donor, stem cell transplants. They found that because healthy red cells live 3-4 months, compared with 3-4 weeks for sickle red cells, complete replacement of the patients’ bone marrow is not necessary. As low as one-in-five of the blood cells in the bone marrow factory coming from healthy donor stem cells may be enough to fix the disease, and this result suggested that reaching a 20% threshold with gene therapy methods might be successful. However, aiming for higher levels of replacement by donor stem cells or genetically modified cells would ensure longevity of these healthier blood stem cells.
So in 2014, Tisdale joined forces with a biotech company, and their collaboration culminated in a gene addition strategy that uses a lentiviral vector to deliver a working copy of the gene encoding for normal hemoglobin into the patient’s bone marrow cells (PMID: 35773052). In a clinical trial leading to its approval, 88% of patients saw their disease manifestations completely resolve.
However, as with the CRISPR approach, the delicate process can have serious side effects. Toxic conditioning protocols such as chemotherapy are necessary to ensure the bone marrow does not reject the modified cells. Then there are the hospital stays to prepare and collect a patient’s stem cells, modify them in a lab, and reinfuse the corrected cells. Those are followed by a lengthy period of medical monitoring to ensure enough healthy hemoglobin continues to be produced.
According to Tisdale, in vivo gene editing might be one way to bring down costs, improve treatment accessibility, and simplify the whole process. Work is underway to encode viral vectors or lipid nanoparticles with antibodies expressed in the bone marrow, which would act as a ZIP Code for their intended destination. The vector could then be administered intravenously, travel directly to the bone marrow, and precisely deliver its gene-correcting payload. It’s not all science fiction: In vivo gene therapy using lipid nanoparticles is currently being used to treat some liver conditions.
“We got Covid vaccines around the globe, basically lipid nanoparticles with RNA inside. This is what we’re trying to make—a lipid nanoparticle with RNA inside to treat sickle cell disease,” said Tisdale, who notes that his collaborators receive funding from the Bill and Melinda Gates Foundation that supports the development of in vivo gene delivery applications.
Another hurdle to overcome is the high expense of producing viral vectors. Tisdale’s group developed a vector that is 10-fold as high in its titer to bring down costs.
Optimizing stem cell transplants
Stem cell treatments have traditionally been reserved for children with SCD who had a sibling donor who was a complete tissue match, a luxury only available to about 15% of patients. Because they have less organ damage than adults with the condition, children are better able to tolerate the rigorous myeloablative conditioning regimen required to make space in the bone marrow and to ensure the donor cells aren’t rejected.
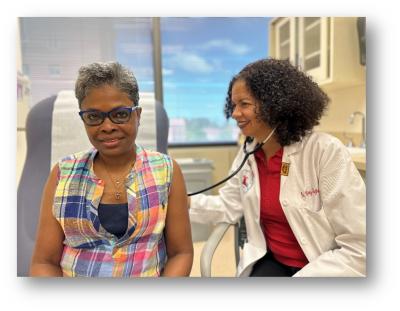
CREDIT: NHLBI
Courtney Fitzhugh working with Tyese Womack, a patient treated for SCD, at the NIH Clinical Center. Womack is now sickle cell disease-free 5.5 years following haploidentical transplant. Fitzhugh’s team is developing new types of stem cell transplants that make the curative procedure possible for a wider range of patients.
By offering stem cell treatments to people who have donors who are a partial genetic match (haploidentical), Courtney Fitzhugh, a Lasker Clinical Research Scholar at NHLBI’s Laboratory of Early Sickle Mortality Prevention, has expanded the possibility of stem cell treatments to 90% of patients with SCD. Furthermore, her group is optimizing lower-intensity conditioning protocols to make the procedure safe for more adults, even those with severe organ damage.
“Curative therapies need to be myriad to meet all the different populations of patients with SCD,” added Emily Limerick, a staff clinician in Fitzhugh’s lab who manages all aspects of patient clinical care at the CC before and after treatment. “Everyone comes with this wondrously optimistic hope of what these curative therapies represent, and the idea of a new beginning is an exciting and pretty universal sentiment among our patients.”
The NIH is one of the originators of low-intensity conditioning protocols, and the Fitzhugh team is exploring using antibodies as a replacement for radiation during the conditioning process. “We think radiation may contribute to some of the complications we see including leukemia, solid tumors, and other short-term complications,” she said. “We’re not just trying to reverse SCD; we want to impact quality of life, prolong survival, and see how different conditioning regimens impact organ function.” Fitzhugh’s team has shown improved heart function (PMID: 37282828) with stable or even improved lung function (PMID: 39189784). The team is about to submit a paper showing preservation of kidney function after lower-intensity conditioning transplants.
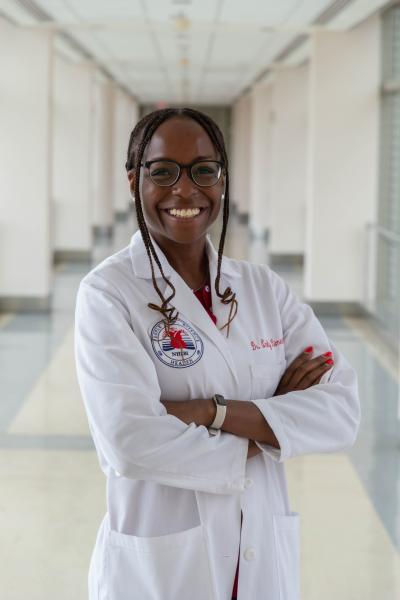
CREDIT: MOHAMED ALI, NHLBI
Emily Limerick manages the comprehensive clinical care of patients being treated for SCD at the NIH Clinical Center. Her patients seeking a cure come from as far away as France or Honduras, and as nearby as Maryland.
Work is also underway to identify genetic markers for future leukemia development after transplant. “If patients have those biomarkers at baseline, we wouldn’t recommend gene therapy because it depends on their own cells versus donor cells,” said Fitzhugh, who is involved in a multicenter study with hundreds of patients to better understand the multifaceted impact of transplants (NCT05153967). “We’ll be comparing children versus adults, transplanted versus not transplanted, high- versus low-intensity conditioning protocols, and gene-therapy strategies versus more traditional types of transplants.”
Back on the floor of the CC, Limerick is optimistic for what the expanding range of curative treatments represent for SCD patients, and she emphasizes the importance of making gene therapies scalable and available to people across the globe who need them most. She’s betting that over time technology will continue its rapid march, and capacity to deliver transformative cures will improve. “These early gene therapies are just the beginning,” Limerick said. “They’re just the tip of the iceberg and there’s a lot more to come.”
NHLBI’s “Today’s Faces of Sickle Cell Disease” highlights stories of people living with sickle cell disease, and the clinicians and researchers whose work offers them hope for a cure.
This page was last updated on Monday, January 6, 2025