How Cells Crawl
Illuminating the Dynamics of Cell Motility
No, Masur Auditorium hadn’t become a campground. That orange dome-shaped tent sitting in the middle of the stage was a prop for a Mider Lecture given as part of the Wednesday Afternoon Lecture Series. Jennifer Lippincott-Schwartz used it to demonstrate what happens to a cell’s “skeleton,” or more precisely its cytoskeleton, when it crawls.
Cells crawl for all sorts of reasons: to form new tissue during embryonic development; to heal wounds; to defend against invading microorganisms; to remodel bone; to regenerate nerves; and more. A cell’s movement is driven by continuous remodeling of the cytoskeleton and is mediated by the lamellipodia (tiny filaments composed of a protein called actin) located at the lamella (front edge of the cell). When actin subunits are added to the lamellipodia in a process known as polymerization, a pushing force is generated. After the polymerization has occurred, the molecular motor, myosin II, is added to cause a contractile force. As the actin filament cytoskeleton pushes and contracts, the cell slowly crawls along.
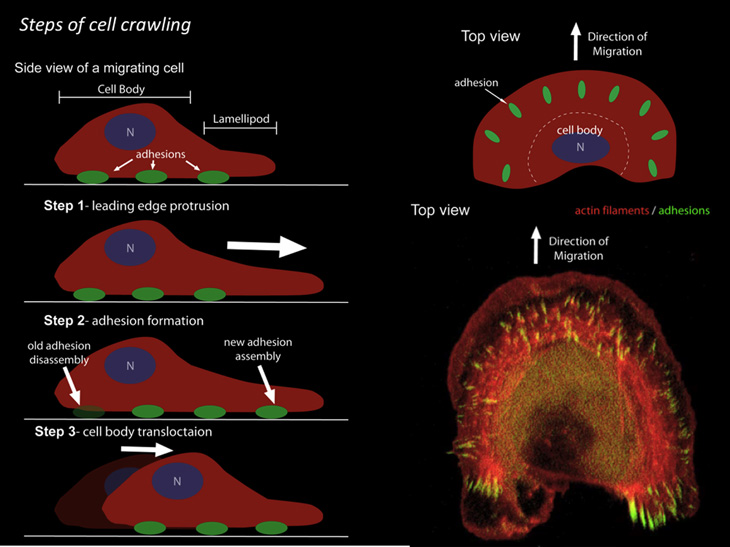
DYLAN BURNETTE, NICHD
To see a video of a crawling cell in motion, go to https://youtu.be/TB6H8RoyPh0.
ERNIE BRANSON
DDIR Michael Gottesman (right) presented Jennifer Lippincott-Schwartz (left) with a framed plaque after the WALS/Mider lecture.
Scientists typically use electron microscopy to get high-resolution, but static, images of the lamellipodia. Conventional confocal imaging can capture live, but blurry, images of cells crawling. Lippincott-Schwartz, a distinguished investigator in the National Institute of Child Health and Human Development (NICHD), has played a leading role in advancing microscopy technologies to allow scientists to see high-resolution images of cells in action.
Her lab helped develop photo-activated localization microscopy (PALM), a technique that uses repeated activation, imaging, and bleaching of photo-switchable fluorescent probes to obtain resolutions of 10 to 20 nanometers, about 10 times the size of an average protein. PALM and other recent super-resolution imaging techniques have ushered a new era of investigations into cellular mechanics.
Lippincott-Schwartz wowed the audience with stunning videos of a live cell crawling. She explained how studies of actin’s continual reorganization at the cell’s lamella have yielded novel insights into the fundamental mechanics of cell motility. She and her NICHD colleagues found that actin arcs of the lamella were the base against which the lamellipodium’s polymerizing actin filaments protrude (Nature Cell Biol 13:371-382, 2011). Furthermore, the high spatial and temporal resolution analysis revealed that actin filaments evolve into the actin arcs as they interact with myosin II, demonstrating a heretofore unknown continuity between the lamellipodia and lamella actin networks.
She described how Dylan Burnette, the leading postdoctoral researcher on the project, studied the spatial relationship between actin and the focal adhesion protein zyxin and found a correlation between the contracting actin arcs and focal adhesion points (actin-rich structures that enable cells to adhere to the extracellular matrix). Moving actin arcs slowed down near newly forming focal adhesions, likened to “feet” at the point of contact between the cell and a surface. This interplay between actin arcs and focal adhesions in turn creates an anchored, stiff substrate against which the polymerizing actin filaments extend the plasma membrane. The combined dynamics of these systems result in the determined crawl of a migrating cell.
Lippincott-Schwartz and Burnette wondered whether this “contractile apparatus” played other roles in a migrating cell’s shape. Burnette visualized the arrangement of actin and myosin throughout the cell three-dimensionally. He saw that myosin II was localized to the actin arcs of a cell’s dorsal side, not to the actin stress fibers connecting the dorsal surface to the ventral focal adhesions. This finding suggests that contractile force is therefore localized to the actin arcs.“What this system really looks like is a tent,” said Lippincott-Schwartz as she walked over to the tent on center stage. The poles represent the actin stress fibers that go from the base to the top of the cell, she explained. As she pushed down on the tent, it flattened just as a crawling cell would.
She hypothesized that such a system underlies the flattening of a cell’s leading edge, allowing the cell to move into the tight spaces of surrounding substrates. Her studies have generated exciting insights into a critical process in developmental biology and cancer metastasis.
Although Lippincott-Schwartz and Burnette have captured astounding images of cellular movement using super-resolution microscopy, they are focused on advancing the mechanistic understanding of cell motility rather than repeatedly imaging the same processes with each new microscopy technique.
After the presentation, Burnette praised Lippincott-Schwartz as a fantastic mentor who has helped him embark on his independent research career, in which he will be continuing his quest to understand cytoskeleton behaviors under a variety of physiological conditions and conditions relevant to disease states.
Meanwhile, Lippincott-Schwartz and her trainees are applying high-resolution imaging techniques to their investigation of the diverse systems of mitochondrial fusion, Golgi organization, glucose uptake, and hepatocyte metabolism. They are poised to make many more great leaps forward in understanding dynamic cellular functions.
The G. Burroughs Mider Lecture, established in 1968, honors the first director of NIH laboratories and clinics. The lecture is presented by an NIH intramural scientist in recognition of outstanding contributions to biomedical research. Lippincott-Schwartz is chief of NICHD’s Section on Organelle Biology in the Cell Biology and Metabolism Branch, a member of the National Academy of Sciences, and president-elect of the American Society of Cell Biology. To view the video of her talk, “Navigating the Cellular Landscape with New Optical Probes, Imaging Strategies, and Technical Innovations,” visit http://videocast.nih.gov/launch.asp?17997.
This page was last updated on Thursday, April 28, 2022