Karl Deisseroth: Optical Deconstruction of Biological Systems
Stanford Neuroscience Pioneer Thrills WALS Audience at Nirenberg Lecture
Karl Deisseroth of Stanford University (Stanford, California) has been changing the face of neuroscience and behavioral research one pioneering technique at a time.
“He has, more than anyone [else] we can point to in the last decade, developed and applied, and then distributed, remarkable technologies to help us understand neuroscience in ways that have been truly enlightening,” said NIH Director Francis Collins in introducing Deisseroth as the speaker at the fourth annual Marshall W. Nirenberg Lecture on June 11, 2014, in Masur Auditorium (Building 10). “Nature, in its article about him about a year ago, called him the ‘Method Man’ because of the way in which he continually comes up with creative approaches that open new windows into understanding how the nervous system works.”
Deisseroth, a Howard Hughes Medical Institute Investigator and professor of bioengineering and of psychiatry and behavioral sciences at Stanford, is the recipient of many awards and is a member of the National Academy of Sciences. It’s fitting that he was invited to give the Nirenberg Lecture because his father at one point worked for the Nobelist.
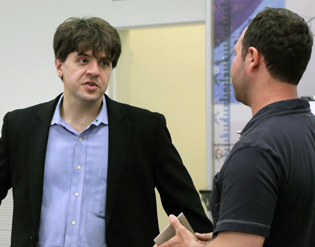
BILL BRANSON
Karl Deisseroth (Stanford) chatted with NIHers after his WALS lecture on the “Optical Deconstruction of Fully Assembled Biological Systems.”
During the lecture, Deisseroth shared results and exciting advances in optogenetics technology; fiber photometry; and CLARITY (which stands for clear, lipid-exchanged, acrylamide-hybridized rigid, imaging/immunostaining–compatible tissue hydrogel), a method his lab developed for keeping three-dimensional tissue intact. His team and others have been using these techniques to map neural networks, discern the molecular identities of cells that are naturally active in the course of behavior, and gain insight into what can go wrong in disease.
Optogenetics combines light and genetically encoded light-sensitive proteins to control cell behavior. At the heart of optogenetics are microbial opsins, light-responsive receptor proteins that can sense light and modulate cell activity. Deisseroth and his colleagues brought optogenetics to the forefront of science in 2005, when they inserted a light-sensitive gene, channelrhodopsin-2 (from pond algae), into selected mammalian neurons and showed that the light pulses could trigger the neurons to fire at their normal speed of a few milliseconds. Although this work was not the first demonstration of a genetically encoded method to gain optical control of neurons, the precise triggering of a single protein on a physiologically relevant timescale overcame significant challenges faced by earlier multicomponent techniques (Nat Neurosci 8:1263–1268, 2005).
In the decade since, Deisseroth’s research has progressed rapidly. Advances in cellular-transduction, gene-targeting, and gene-expression technologies have facilitated the selective targeting of opsins to certain neurons in the mouse brain. His lab developed a fiber-optic neural interface to both control and distinguish between patterns of activity that contribute to motivated behavior, reward learning, and anxiety. Because Deisseroth is a psychiatrist who focuses on treatment-resistant depression, he is particularly interested in the neural-circuit underpinnings of these behaviors.
In addition to artificially controlling neural activity, Deisseroth’s group used fiber photometry to directly observe, and optically record in real time, neural activity in free-moving animals, illustrating a direct link to behavior during social interactions (Cell 157:1535–1551, 2014).
Perhaps one of the most important technologies that Deisseroth’s group devised is CLARITY, a method to make the whole brain transparent so it could be easily imaged (Nat Methods 106:508–513, 2013). Before CLARITY, scientists had to reconstruct three-dimensional images from slices of neural tissue, because imaging an entire brain was impossible: The lipid layers that surround the cells obscure the view.
Deisseroth’s team figured out a way to remove the lipids without disrupting the rest of the brain structure. They created a meshlike hydrogel to hold the other components in place and then incubated the brain in detergent to solubilize lipids. Once the fat is removed, the brain is transparent—and able to be more easily imaged—as well as permeable to macromolecules, which facilitates molecular phenotyping of cells.
CLARITY allows high-resolution imaging of very fine cellular structures, such as axons. Deisseroth further refined the method using light-sheet microscopy to illuminate only the region of the tissue being imaged at a particular time (Nat Protoc 9:1682–1697, 2014). When combined with commercially available CLARITY-optimized microscope objectives, both the speed and the image quality of tissue images have been greatly enhanced.
Deisseroth enthusiastically shares the tools with the scientific community. His lab hosts training courses and maintains CLARITY resources (http://clarityresourcecenter.org) and Wiki Web sites, enabling two-way sharing of protocols, instructions, and feedback from other users of the technology. The end result is an ever widening field of scientists using optognetics to help elucidate the inner workings of the brain, which will undoubtedly lead to significant impacts not only on basic research, but also on the treatment of psychiatric and neurodegenerative diseases.
The Nirenberg Lecture commemorates the late Marshall Nirenberg, who shared the Nobel Prize for Physiology or Medicine in 1968 for deciphering the genetic code. To watch a videocast of Karl Deisseroth’s June 11, 2014, lecture, entitled “Optical Deconstruction of Fully Assembled Biological Systems,” go to http://videocast.nih.gov/launch.asp?18552.
This page was last updated on Tuesday, April 26, 2022