Creating a “Spellchecker” for Genes
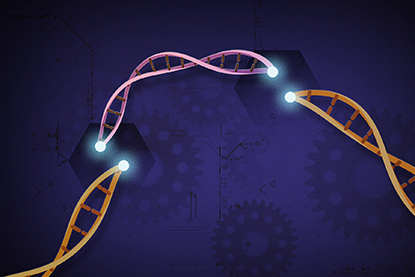
CREDIT: ERNESTO DEL AGUILA III, NHGRI
CRISPR-Cas9 is a customizable tool that lets scientists cut and insert small pieces of DNA at precise areas along a DNA strand. Lothar Hennighausen’s team has led the way in describing the extent of unwanted molecular scarring that the CRISPR-Cas9 technique can cause at target sites of mammalian genomes.
Imagine a pair of molecular scissors that could snip and tweak sections of the genetic alphabet. Or tweezers that could pluck one letter and replace it with another. These tools have become a reality in recent years, allowing scientists to correct “misspellings” in the genetic code.
“Gene-editing technologies have taken center stage in biomedical research, with boundless impact on translational medicine,” said Lothar Hennighausen, chief of the Laboratory of Genetics and Physiology in the National Institute of Diabetes and Digestive and Kidney Diseases (NIDDK).
CRISPR, which stands for “Clustered, Regularly Interspaced, Short Palindromic Repeat,” together with CRISPR-associated 9 (CAS9), has emerged as one of the most promising gene-editing tools to study disease. Known for its efficiency and ease of use, the CRISPR-CAS9 (hereafter CRISPR) gene-editing method works by making cuts at targeted sites on a genome, altering an organism’s DNA.
Hennighausen’s lab has embraced this budding technology and examined the mouse genome (Nucleic Acids Res 44:1052–1063, 2016; DOI:10.1093/nar/gkv999). In collaboration with Chengyu Liu—the director of National Heart, Lung, and Blood Institute’s Transgenic Core facility—the team went on to make key discoveries in gene editing. In 2017, they led the way in describing the extent of unwanted molecular scarring that the CRISPR technique can cause at target sites of mammalian genomes. By mutating 17 genomic sites and analyzing over 600 mice, the researchers discovered large, unintended DNA deletions and insertions associated with the CRISPR method (Nat Commun 8:Article number 15464, 2017). Other scientists have since published similar findings on the random insertions and deletions that the technique can cause.
But what about the CRISPR method’s effects outside the sites targeted for mutations? In October 2018, with support from the Deputy Director for Intramural Research Innovation Awards program, Hennighausen’s team published a paper on the frequency of new mutations in CRISPR-method-edited mammalian genomes. Using whole-genome sequencing, which reveals an organism’s complete DNA makeup, the researchers concluded that CRISPR did not cause unexpected off-target damage throughout the genome (Nat Methods 15:756–758, 2018; DOI:10.1038/s41592-018-0148-2).
“This finding was important, as research must address the safety aspects of CRISPR and other new technologies,” said lead author Michaela Willi, a postdoctoral researcher in Hennighausen’s lab.
More recently, Hennighausen’s team used deaminase-base editing, a newer form of gene editing, to introduce mutations in the mouse genome. Most known human diseases are caused by mutations in just one of the four letters, or bases, that make up DNA. Base editors can correct these mutations by converting one base pair into another. Unlike conventional CRISPR technology, base editing does not cut through the DNA, reducing the chance for unwanted errors.
“The exceptional precision of base editors brings fresh air into the genome-engineering toolbox and allows us to avoid problems encountered with conventional CRISPR” techniques, said Hennighausen.
With researchers from the NHLBI, the Broad Institute, and Harvard University, Hennighausen’s team explored the fidelity, or accuracy and reliability, of deaminase-base editing in the mouse genome. The study, published in November 2018, found that the two classes of base editors—cytosine base editors (CBE) and adenine base editors (ABE)—had different fidelities. ABEs were more accurate and caused no unintended errors (Nat Commun 9:Article number 4804, 2018; DOI:10.1038/s41467-018-07322-7).
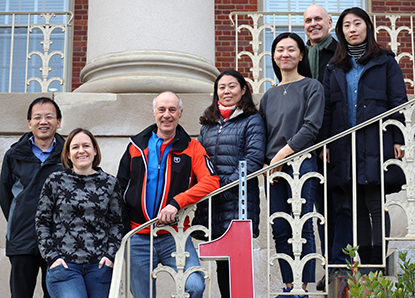
CREDIT: JENNIFER RYMARUK, NIDDK
Lothar Hennighausen’s team has made key discoveries in gene editing. Some of the team members gathered recently for a group photo on the steps of Building One. From left: Chengyu Liu, Michaela Willi, Hennighausen, Xianke Zeng, Hye Kyung (HK) Lee, Harold Smith (director of the NIDDK Genomics Core), and Sojung Kim.
Hennighausen’s team also applied base editing to linked mutations—two or more mutations at different sites in the same chromosome. Generating linked mutations through gene editing could correct certain genetic diseases. The team’s recently published research showed that CBEs can accurately introduce linked mutations into mouse embryos without causing unwanted deletions at target sites (Sci Rep, 2018; DOI:10.1038/s41598-018-33533-5; in press).
“Base editing can correct about 60 percent of known disease-causing mutations,” said Hye Kyung Lee, another postdoctoral researcher in Hennighausen’s lab and a lead author on both base-editing papers. “A thorough understanding of its fidelity is thus critical when considering this technology for human gene therapy.”
Other NIDDK scientists are also applying gene editing to the study of human disease. For example, Andy Golden in NIDDK’s Laboratory of Biochemistry and Genetics uses the CRISPR method to generate mutations in a worm species that shares many genes with humans. By studying how these mutations disrupt gene functioning, the scientists hope to identify potential targets for therapy.
In addition, NIDDK’s Genetics of Development and Disease Branch, led by Richard Proia, together with NIDDK staff scientist Maria Laura Allende and Cynthia Tifft of the National Human Genome Research Institute’s Medical Genetics Branch, used gene editing in their recent publication on Sandhoff disease, a deadly childhood genetic disorder. Using the CRISPR method, the researchers created healthy stem cells to form cerebral organoids, miniature organs that mimic the brain, and compared them with Sandhoff-affected organoids, gaining new insights into the disease’s progression (J Lipid Res 59:550–563, 2018; DOI:10.1194/jlr.M081323).
The Proia and Tifft labs are using the CRISPR method and base editing in several current projects, such as correcting point mutations in cells from patients with late-onset Tay-Sachs disease, a neurodegenerative disorder that affects people in their 20s and 30s.
But as much promise as these gene-editing tools hold for preventing and treating disease, there are ethical issues to be considered, too. Recently, a Chinese scientist reported that he used CRISPR editing on human embryos to disable a particular gene. Scientists worldwide expressed their outrage over the scientist’s willingness to flout international ethics norms. In a November 28, 2018, statement, NIH Director Francis S. Collins called for the “development of binding international consensus on setting limits for this kind of research,” without which, the world could see a flood of “ill-considered and unethical projects.”
Applying gene-editing technologies to human health requires a full understanding of their mechanisms and potential negative consequences as well as thoughtful discussion and consideration of ethical implications. While there is still a long way to go, when used responsibly, the CRISPR method and base-editing tools could pave a key pathway to benefit public health.
This page was last updated on Tuesday, April 5, 2022