Drs. Natasha Caplen and Richard Maraia — What's Next in the RNAge?
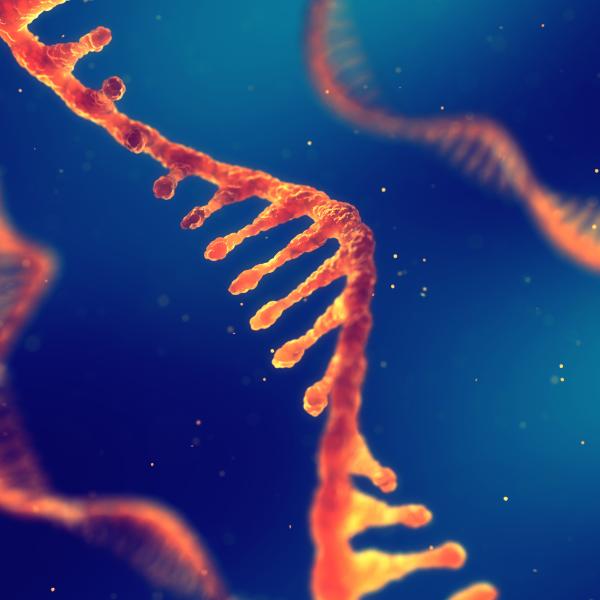
The success of mRNA vaccines against the coronavirus that causes COVID-19 has brought RNA biology into the limelight. Now, with the world's attention, what's next for this biomedical rising star? NIH scientists, Dr. Richard Maraia and Dr. Natasha Caplen, have long recognized the potential of RNA in improving human health. In this episode, they discuss the prospects of RNA biology and how their work could inform the future of RNA as an innovative class of medicine.
Categories:
RNA Microbiology and infectious disease Vaccines Structural biology
Transcript
>> Diego (narration): Three letters have been on everyone’s lips for quite some time now.
>> Newsclips: RNA, RNA, RNA
>> Diego (narration): If there was an award for the most influential acronym of the year, RNA would surely make this year’s shortlist.
>> Clip from the film “The Mask”: You love me, you really love me. Thank you.
>> Diego (narration): Now, add another letter, m, and what do you get? The special sauce in Moderna and Pfizer’s vaccines against the SARS-CoV-2 virus that causes COVID-19.
>> Stephen Hoge: At Moderna, we believe messenger RNA represents unprecedented opportunity to develop medicine for patients, one that could have a dramatic impact on the course of human health and disease.
>> Diego (narration): That’s how Stephen Hoge, the president of Moderna, talked about the promise of RNA biology before it was really put to the test during the pandemic. Lucky for us the mRNA platform for vaccines has aced the test, the final exam, it’s gotten its degree and it’s waving to the audience as valedictorian of its class.
>> Clip from “Pomp and Circumstance”
>> Diego (narration): But now that the spotlight is shining down RNA biology, what’s next for the biomedical rising star?
In this episode, I talk to two scientist who have long recognized the potential of RNA in improving human health and whose work could inform the future of RNA as an innovative class of medicine.
Before we go any farther, we have to talk about what RNA actually is and how it works. For starters, it stands for ribonucleic acid, which means it’s made of molecular building blocks called nucleotides, similar to those that make up its scene-stealing sibling, deoxyribonucleic acid, better known as DNA. But there are some key differences between the DNA and RNA that make the latter a little bit more difficult to understand but arguably more intriguing.
>> Dr. Maraia: DNA just stores the information. The stuff that actually does the work is the RNA.
>> Diego (narration): That’s Dr. Richard Maraia.
>> Dr. Maraia: The RNA is not just simply a copy of the DNA. It’s much more complex.
>> Diego (narration): A senior investigator, and head of the section on molecular and cell biology at the National Institute on Child Health and Human Development, Dr. Maraia studies how RNAs regulate the flow of genetic information inside the cell. In the study of life, the central dogma of molecular biology explains how our genetic code—that’s the nucleotides of DNA represented by the letters A, T, C, and G—are decrypted to the make proteins, the actual workhorses of the cell that carry out the many functions necessary for life. Basically, it says that for genes to be expressed, DNA must first be transcribed into RNA which can then be translated into the proteins. But as Dr. Maraia says, the process of making RNA from DNA isn’t a simple copy-paste.
>> Dr. Maraia: RNA gets spliced. You know, 99% of the genes that translate the two-dimensional genetic code into a three-dimensional organism gets spliced, and so the contiguous, linear DNA gets chopped up and then put together and then out comes an organism.
>> Diego: Splicing is the way cells cut out parts of a gene that aren’t necessary to produce their corresponding proteins. It’s the cell’s discerning film editor. If DNA is the raw footage of a documentary, with its extended scenes and B-roll, then RNA is the final theatrical version that goes up on the silver screen. The original footage, again the DNA, is stashed safely away in the filmmaker’s vault—that’d be the nucleus of the cell—while the edited or spliced version includes just the right information to relay movie’s message—hence why this type of RNA is called messenger RNA or mRNA.
If splicing weren’t a thing, it’d be like listening to a podcast where the host just drones on and on about who knows what, meandering from one thought to the next, including unnecessary details, belaboring the point, and not knowing when to stop…you get the point. We need edits, in the real world and in the cell.
So, mRNA transcripts that result from splicing are the first fully intact working copy of the information of life. Because they don’t permanently change the genome, break down over time, and exploit the cell’s own machinery to make proteins, mRNAs can be used to deliver missing or new proteins into a biological system. Which is exactly what Moderna did with the spike protein from which the coronavirus gets is name.
MRNAs can be used to add new or missing genes, but they can also be targeted to prevent certain genes from being expressed. It’s what’s called RNA interference or RNAi, and it was co-discovered in mammalian cells by Dr. Natasha Caplen over 20 years ago.
>> Dr. Caplen: We found that very small RNA molecules, these are molecules that are actually only consisting of about 22 nucleotides, they are manipulating and altering gene expression all the time.
>> Diego (narration): Dr. Caplen is an investigator and head of the Functional Genetics Section at Center for Cancer Research in the National Cancer Institute. She recently published a review on the history of RNAi, which was first observed in the 90s by two scientists trying to manipulate the color of petunias. They added RNA copies of a gene responsible for the purple pigment in the flowers’ petals thinking that the petunias would be a deeper violet. Instead…
>> Dr. Caplen: …it silenced the genes.
>>Diego (narration): The blooms were white, not purple.
>> Dr. Caplen: But they had no idea why. It was truly a phenomenon. It was like we see this, but we don't know why. And people were plugging away trying to come up with ideas. It wasn't until the studies by Craig Mello and Andy Fire in C. elegans that they realized it was double-stranded RNA.
>> Diego (narration): As it turns out, when you introduce a short sequence of RNA that perfectly complements an existing mRNA in the cell, it sets off molecular machinery that will eliminate the mRNA altogether. So, in the chain from DNA to protein, the process of RNAi quite literally kills the messenger.
>> Dr. Caplen: So then, you get the peak of studies looking at it, proving that it occurs in Drosophila. And then, the next breakthrough, which I was part of, was proving that it occurred in the mammalian cells. And that became absolutely a revolution. Within months we had a whole new mechanism for regulating gene expression and a whole new way at looking at gene function, that we had never been able to do mammalian cells up until then.
>> Diego (narration): Today, many labs across the world are working to harness the power of RNAi to directly silence specific genes linked to a particular disease. But Dr. Caplen has leveraged the technology to search for clues in a trickier puzzle.
>> Dr. Caplen: For many years, my laboratory has focused on how RNA molecules are involved in different aspects of cancer biology. What we were able to find out over many years of research was that we could actually mimic those small RNAs, and we could use that to explore how genes work under normal circumstances and then when it goes wrong in a cancer.
>> Diego (interview): I see. What type of cancer are we talking about?
>> Dr. Caplen: So, one of the projects that I became involved with a few years ago started out as a collaboration with a team in the pediatric oncology branch at the National Cancer Institute. What they were interested in was understanding more about a tumor called Ewing sarcoma that effects predominantly children through young adolescents, and these are tumors that arise in the bone and soft tissues. It's very rare. Unfortunately, at this point in time, the treatments that we can offer tend to be very aggressive. There's a risk, therefore, of some secondary effects later in life, and there's some individuals that, unfortunately, we really have very few options in time to treat.
>> Diego (interview): Mm-hmm. And where does RNA biology come into play in this type of cancer?
>> Dr. Caplen: So, in my prior research experience studying small RNAs, we knew that we could use the approaches and the technologies that we had developed to maybe understand a little bit more about the biology of this tumor type. So, I worked with members of the pediatric oncology branch and a team at the National Center for Advancing Translational Sciences, or NCATS, to build what we call an RNA interference screen. So, this is where we can take small RNA molecules that actually correspond to every single transcript, every single mRNA, that makes a protein in the entire human genome.
Now when you do screens, we have no idea often what the answer is going to be. By definition, when we're doing a screen, we're often asking a very open-ended question. We might think we can prioritize or, preferably, hypothesize about what we will get from it, but we can't guarantee that. But what we discovered, as a consequence of the findings, is that in a subset, so, in a small group that represents about 40% of the individuals that are going to be diagnosed with Ewing sarcoma in any one year, that the DNA breaks—if that happens in a very specific place, you get a piece of DNA from one gene and a piece of DNA from another gene. That's going to go on and make this very aberrant protein, that’s called a fusion protein. But in between those two steps is the RNA. And in this particular case, the RNA has to remove one particular piece of RNA, or it won't make the protein that drives these tumors. So, we discovered is that there’s an RNA-binding protein that's required for that particular RNA processing event, it’s a splicing event.
>> Diego (narration): In other words, the irregular mRNA transcript copied from the broken DNA, must be spliced by a separate protein or it won’t go on to make the fusion protein that causes the cancer. So, if you want to stop the cancer, you could stop the splicing protein from editing the fusion transcript. But…
>> Dr. Caplen: …how that works, how it does that, is less known. And so, that's actually what my lab is currently working on; studying the biophyiscs, the physical interactions of this RNA-binding protein with our RNA of interest. The idea is if we can understand exactly how that interaction works, we might be able to find a way of actually inhibiting just that very specific interaction.
>> Diego (narration): That interaction depends on the structure. One of the key tenets in the study of life is that structure determines function. Meaning that the way something is designed enables it to fulfill its job within an organism. That applies at the physiological level—think the four chambers of the heart or how our joints are put together—and scales down to the molecular level. For a protein to bind to its target, the two have to fit perfectly, like a key in the correct lock. A misshapen protein can’t do its job any more than your car key can open the front door to your house.
And the same can be said for RNAs.
>> Dr. Caplen: If there was an area where we're still trying to learn a lot and are thinking through, it is the impact of structure on how RNAs work. When we look at a molecular biology textbook, it'll show an RNA molecule, and it's often shown as just a line, but they have complex structures that are flexible, and they're covered in proteins. And so, that is one of the things we’ve been trying to think about: What is actually happening in the cell, in terms of RNA structure?
>> Diego (narration): So far, we’ve mostly been talking about RNAs as transcripts for proteins. But the RNA family tree has several branches, each with its own type of structure and therefore purpose.
>> Dr. Maraia: There’s a lot of RNAs out there. There's ribosomal RNA. There's transfer RNA. There's small-nuclear RNA that are involved in the splicing reaction itself.
>> Diego (narration): That’s Dr. Maraia again.
>> Dr. Maraia: And then there's all kinds of other RNAs that help proteins get to the right place in the cell and all that stuff. Okay, but many of those RNAs have what's called post-transcriptional chemical modifications. Those modifications work a lot of different ways. Many of them, affect how RNA folds into different forms.
>> Diego (narration): In RNA origami, post-transcriptional modifications are the instructions that turns a flat, two-dimensional piece of paper, into an intricate three-dimensional crane. Dr. Maraia says that if you’re only looking at the sequence of bases in an RNA, you’re only getting fraction of the story.
>> Dr. Maraia: There are over nearly 150 different modifications that are known to exist. Imagine the complexity of all of that stuff. That's a whole another level of information.
The best, probably, known example is tRNA, or I should say, are the different tRNAs.
>> Diego (narration): TRNAs are adaptor molecules that match the nucleotide sequence of mRNAs with the right amino acids to make protein. To the untrained eye, they might look like random clumps of lint, but their form is very specific.
>> Dr. Maraia: They have to form this three-dimensional -- well, cloverleaf is a two dimensional, but in three dimensional they form another thing that actually looks more like an L-shaped thing. It has to fit perfectly in the ribosome, and it has to be the adapter that delivers amino acids in the right way. They’re not just linear things. I mean, it's not just free game, any tRNA can go there, or else you'd get the wrong amino acid, and it'd be a big mess.
>> Diego (interview): I believe it.
>> Dr. Maraia: We'd just be a bowl of jelly. So, modifications basically control it.
>> Diego (interview): Gotcha, so these modifications tightly regulate the shape of RNAs and consequently how they function, but I would imagine that there are instances where the quality control slips up, no?
>> Dr. Maraia: Absolutely. There are hundreds of diseases that are attributable to lack of modifications in RNA. We referred to them now as “RNA modopathies.” They're modification -opathies.
>> Diego (interview): Gotcha.
>> Dr. Maraia: Most of the diseases that are known to result from deficiencies of RNA modification are from deficiencies in tRNA modification. And the reason why that is, is because things come to the surface where you look. There's an old joke about two guys who are outside a pub late at night, and they were standing under a streetlight, and you know, they're a little tipsy. And they're fumbling around, looking around, and some other guys came out and said, "Well, what are you doing?" They say, "Well, we're looking for the car keys. We want to get home." The guy who came out said, "Well, how long have you been here?" They say, "Oh, about 20 minutes. We still haven't found them." "Well, where's your car?" "Oh, my car is two blocks down." "Well, maybe you lost them over there." And they said, "Why are looking here?" They said, "Well, this is the only place where the light is."
>> Diego (interview): [Laughter] Right.
>> Dr. Maraia: So, anyway, the reason why most of the diseases are in tRNA modification is because tRNAs are the most heavily modified RNAs that we know of.
>>Diego (interview): Well now I’m wondering, since you’re saying modifications effect all kinds of RNAs, are they also crucial for mRNAs?
>> Dr. Maraia: Yes. Messenger are know to carry a lot of modifications. So, you know, the big thing that allowed development of the mRNA-based vaccine was to put the right modifications on it. It was absolutely essential. Without that, it wouldn’t have been possible.
>> Diego (interview): I had no idea.
>> Dr. Maraia: We use modification in present-day humans, to distinguish an invader from ourselves. And so, Moderna had to put modification, especially pseudouridine, all throughout the COVID or the SARS-CoV-2 mRNA, in order for it not to provoke the immune system in a bad way. If pseudouridine wasn't on the vaccine RNA, it would be recognized as invader, and it would set off the whole cytokine storm in and of itself. So, delivering the RNA and injecting it into someone without modifications doesn't work, flat out no. It's a no starter
>> Diego (narration): The success of mRNA vaccines with their indispensable modifications, opens the door to the possibilities of RNA therapeutics. To realize the full potential of RNA in treating or preventing disease though, scientist will have to get a clearer picture of what’s actually happening at the single-molecule level.
Earlier this year, Dr. Maraia co-authored a commentary in Nature Genetics calling for the resources and infrastructure that would make it possible to sequence full-length RNAs the way the Human Genome Project mapped out all the genes in our DNA.
>> Dr. Maraia: We need new technology like that was developed for the genome project 30 year ago, to be able to directly determine the modifications on RNAs.
>> Diego (narration): The Human Genome Project gave us the complete genetic blueprint for building a human being, but the blueprint didn’t include instruction on how to translate DNA into flesh and bone. A Human RNA Project could help interpret the blueprints and fill gaps in our understanding of how the nanoscopic scale up to affect health and disease— maybe even find ways to turn mystery into medicine and biology into technology.
>> Dr. Maraia: We don't know where it's going to go. Remember when PCR was invented in the early 1990s? It swept through the community, and people started doing it like crazy. Every week, you'd just, literally, walk through the halls of NIH, and people had a new invention they could do with PCR. It was amazing. And then, that happened again with the luminous sequence. They just invent new things you can do. You wake up in the morning and realize, oh, I just dreamed of another way, another thing I can do. And I think the same is going to happen.
>> Diego (narration): It's a shame that it took the urgency of a pandemic for RNA biology to take center stage. Perhaps the only silver lining is that we’ve now seen it’s biomedical force in action and are poised to capitalize on the decades of research that have shown it to be a molecular swiss army knife. If RNA can selectively silence genes, introduce antigens in vaccines, and serve to conduct cancer research, imagine what other tools it could produce if we dedicate the same attention to it when it’s not a global emergency.
>> Dr. Caplen: In the last year and a half, everyone's become very familiar with the concept of viral RNAs and we are now seeing that it is a targetable molecule, and it's a molecule that has interventional properties that two years ago, many of us who were in the RNA biology community or have been in the nucleic acid therapeutics community thought would happen. But to see it so rapidly change the face of science in such a short period of time, I think we all agree now that this is the time for us to be able to say RNA needs the resources and the imagination to be able to say now we need to understand how this truly works, so that we can build upon what we have learned for the last 18 months.
Related Episodes
This page was last updated on Tuesday, October 1, 2024