Dr. John Hanover — The Bittersweet Study of Glycobiology
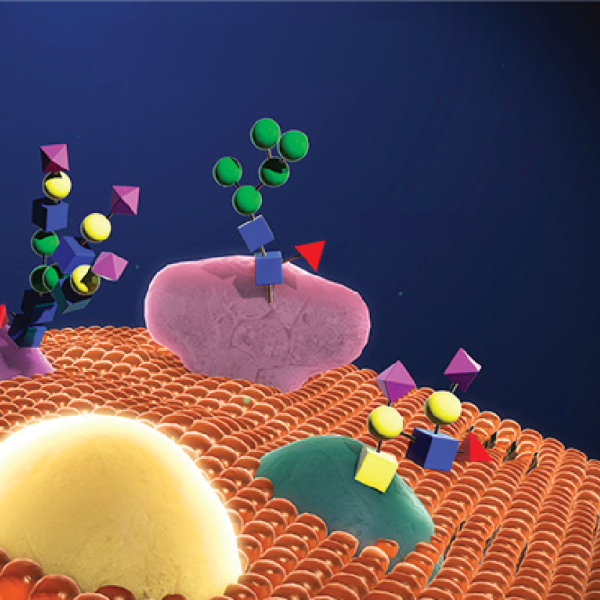
Sugars, also referred to as carbohydrates, aren’t just substances we add to make coffee taste less bitter or food sweeter; they are an entire class of molecules necessary for life. The study of these carbohydrates is called glycobiology. Dr. John Hanover is a glycobiologist and the chief of the laboratory of cell and molecular biology at the National Institute of Diabetes and Digestive and Kidney Diseases (NIDDK). His work is advancing scientists’ understanding of the sugar structures responsible for rare diseases and genetic transgenerational inheritance.
Learn more about Dr. Hanover's research at https://irp.nih.gov/pi/john-hanover.
Categories:
Rare diseases Diabetes Epigenetics
Transcript
>> Diego (narration): When I was in grad school for science communication, there was a story we discussed that really stuck with me. It asked the question, “What would you do if your child has a condition that is new to science?” and it was about a young boy named Bertrand, who had fallen ill with a bevy of neurological and metabolic symptoms.
[PENSIVE MUSIC]
He trembled constantly, had intellectual disabilities, and stared off into space making it hard for him to engaging with others. His eyes didn’t produce any tears so every time he blinked it was like sandpaper scrapped against his corneas [SANDPAPER SOUND EFFECT]. All that on top of life-threatening seizures that had Bertrand in and out of hospitals [FADE IN AMBULANCE SIREN AND HEART MONITORS SOUNDS], where doctors and specialists couldn’t pinpoint the cause for his condition. [FADE OUT].
Until one day, genetic testing revealed he had inherited two mutated copies of a gene that compromised how sugar molecules are removed from defective proteins to signal their disposal. In other words, with these sugar tags stuck in place, Bertrand’s body struggled to recognize and recycle molecular waste, conceivably clogging up the gears of his cells’ machinery, and finally explaining his complications.
Now, you might think of sugars as the substances we add to make food taste sweeter or coffee less bitter; but sugars, also referred to as carbohydrates, are a whole class of molecules that carry out different functions and are necessary for life. The study of these sugar molecules is called glycobiology.
>> Dr. Hanover: Glycobiology contains really what we now call "carbohydrate metabolism."
>> Diego (narration): That’s Dr. John Hanover, he’s glycobiologist and the chief of the laboratory of cell and molecular biology at the National Institute of Diabetes and Digestive and Kidney Diseases.
>> Dr. Hanover: Most of the work that we do and others in the glycobiology field do relate to what are known as "complex glycans."
>> Diego (narration): Dr. Hanover describes complex glycans as larger, chain-like carbohydrate structures made up of different sugar subunits.
>> Dr. Hanover: Glycobiologists generally are interested in these complex glycans because they're so important for physiology, for immunology.
>> Diego (narration): One example of how crucial these glycan molecules are can be seen in our blood.
>> Dr. Hanover: If you're blood group type A, you have a specific glycan structure on your cells, particularly the immune cells and red blood cells. Similarly, the O blood type, which is so-called "universal donor," and the AB, blood type, which is a universal acceptor; these all have different glycan structures that allow the immune system to tolerate the presence of these glycans. So, our bodies are really geared to both recognize and avoid interference from these glycans.
>> Diego (narration): Besides acting like signposts for cellular communications, glycans can also modify proteins and help them fold into the correct shape.
If you tuned into our episode on protein folding with Dr. Lauren Porter, you’ll remember that a protein’s structure determines its function. And in some cases, glycans are tacked on to proteins to stabilize that structure. So, without these modifications, many proteins, like those make up the pores that direct traffic [TRAFFIC SOUNDS] in and out of a cell’s nucleus which are of particular interest to Dr. Hanover, can’t do their jobs in the cell [CAR HORNS].
Glycobiology is a rapidly expanding field. It even took home the Nobel prize in Chemistry last year. The more scientists dig, the more they find that the roots of carbohydrate biology interconnect with all sorts of life’s processes, like epigenetics and embryonic development, reminding us to appreciate the amazing complexity and synchrony it takes to keep us healthy. And in cases of rare genetic disorders, like Bertrand, the domino effect sugar molecules can have, highlight the importance of studying these modifications.
>> Dr. Hanover: There's a very large number of human diseases now, rare human diseases called the congenital disorders of glycosylation. Those present often as neurologic defects. There’s also defects that lead to Alzheimer's, changes in cancer metabolism, and so it's quite broad. Generally speaking, I would say that those defects do not lead to lethality, but they do present often as developmental abnormalities.
>> Diego (narration): In the context of what can be such devastating circumstances, Dr. Hanover is working to advance our understanding of glycans in hopes that it will lead science one step closer to treatments and relief for patients suffering from these conditions. Stayed tuned to hear how he’s breaking down these fundamental sugar structures in the bittersweet study of glycobiology.
[TRANSITION MUSIC]
>> Diego (interview): So, Dr. Hanover within the field of glycobiology you study a specific modification called—I’m probably going to butcher saying this: O-GlcNAsylation.
>> Dr. Hanover: Yeah, O-GlcNAcylation, we call it.
>> Diego (interview): Ok.
>> Dr. Hanover: And Gerry Hart and I, who were sort of the founders of this field, came up with that. This thing is actually O-N-acetylglucosaminylation, very long and cumbersome. So Gerry and I just decided very early on to call it "O-GlcNAc" and O-GlcNAcylation.
>> Diego (interview): Right, ok, O-GlcNAcylation. So what’s the story with O-GlcNAcylation? How did you come across it and what makes it so special?
>> Dr. Hanover: So Gerry and I, with a student of Gerry Hart’s called Carmen Torres Rosa, found that unlike other glycans, this was not found on the cell surface, but it was associated with the nucleus, in particular, nuclear pore complex, and the cytosol.
At the time, and to some extent, even to this day, the glycobiology community was so focused on the cell surface that when we first encountered this, people didn't think that it was true. They thought that nuclear glycosylation was very strange. People really didn't want to accept it.
And, you know, at the time that work was done, no one knew what the nuclear pore was composed of and we were fortunate enough to clone the first of the nucleoporins. I jokingly tell people that we cloned the first nucleoporin so we'd have a substrate for the O-GlcNAc transferase, and it was really actually kind of true. The nucleoporins then became a sort of growth industry. And so the nucleoporins are among the most heavily modified by the O-GlcNAc modification.
>> Diego (interview): So it sounds like O-GLcNAc was pretty elusive. Is that why you’ve referred to it in the past as the dark matter of cellular biology?
>> Dr. Hanover: So, when I say O-GlcNAc is biology's dark matter, in a sense it's a modification that was hiding in plain sight. It was so abundant and so omnipresent on these proteins that people had largely ignored it. A, they couldn't detect it, and B, it was very hard to study
So in addition to the nucleoporins, we've recently found that O-GlcNAc is a key regulator of the DNA damage response. We've also recently shown that it's a key modulator of the DNA methylation that accompanies the early development, particularly those changes in DNA structure that lead to epigenetic inheritance.
When we look at epigenetics, and we have, very extensively, using genetic tools, we find that O-GlcNAc is everywhere. It appears to modify the transcription cycle, it is involved in the so-called "hox genes," or homeobox genes, that occur during developmental time.
>> Diego (interview): So it sounds like O-GlcNAC is really keeping a lot, if not everything, working properly at the cellular level. So I imagine if it glitches, the consequences could be easily seen at the physiological level. Is that something you’ve worked on: narrowing down and maybe even treating disorders that result from mishaps with O-GlcNAc?
>> Dr. Hanover: Yeah, we've done a lot of that. We've worked with some of the intramural colleagues on development of probes for O-GlcNAc in the brain, which allow you to visualize, for example, where these enzymes reside in the brain, and we've recently published that work. That was a wonderful collaboration. The other kind of work we do is associating with physiologists using genetic tools in the mouse to identify what this pathway, what this modification does in heart disease. And we published several papers on that. We're very interested in the vasculature and how it affects the vasculature. And so, once again, I want to emphasize that O-GlcNAc is sort of omnipresent, and so, you know, defects in that pathway have very deleterious effects. There's a form of X-linked intellectual disability where O-GlcNAc transferase itself is actually mutated. It is not a complete loss of function. That is, the enzyme still works, but it has lost some of its ability to recognize substrates appropriately. And these kids have intellectual disability, which is clear evidence that this pathway is directly involved in human disease; potentially such diseases as autism in addition to the neurodegenerative diseases we discussed.
>> Diego (interview): Wow, so there’s no doubt the pathway really is essential. And naturally I’d assume there are different enzymes that regulate the pathway. Ones that add O-GlcNAc and ones that remove it. So it’s not like you can study what would happen if you genetically knocked out one of the enzymes involved in O-GlcNAcylation in mice per se, because the embryo wouldn’t even develop. Is that right?
>> Dr. Hanover: It's a very interesting perspective, Diego. So, what we find is that invertebrates, particularly in the mice where we can do the study, knocking out OGT, the embryos died about embryonic day 5.5, which is very early in development. The O-GlcNAcase survived, but they died perinatally. So, if you remove the enzyme that cleaves O-GlcNAc, they live until right around birth and then they die. And so, it is an essential modification in invertebrates. Interestingly, the O-GlcNAc transferase is essential in the fruit fly, Drosophila, but the O-GlcNAcase is not essential. And then when we move to C. elegans, which is the other system we study, neither enzyme is essential. So even though the pathway is conserved all the way from the nematode C. elegans to man, there's a gradient of importance, and in invertebrates, this extension of the modification to control such things as DNA damage repair and DNA methylation we think has even more profound implications for human disease. Because it's not just human disease associated with an individual, but also the potential for transgenerational inheritance of disease susceptibility.
>> Diego (interview): Interesting. Before we move on, maybe you can help me clarify this a little bit and make sure I'm understanding this correctly. So in the context of epigenetics, DNA methylation is a chemical process that alters the way the genes coded by our DNA are expressed—it doesn’t actually alter the genes just how they’re read. And those chemical changes can be passed down from parent to child. But you’re saying O-GlcNAc is one step before that? That this pathway is responsible for sensing the environment and integrating that information in the cell which then dictates how those methylation changes will occur?
>> Dr. Hanover: That's exactly right. And we've shown that much of this occurs. What we see are tremendous and wholesale changes in DNA methylation.
And so, it's poised, the modification is poised really both as a sensor but also as a mediator of changes in DNA methylation. We're very excited about this because it really is a paradigm shift in the way we think about susceptibility to human disease.
>> Diego (interview): Can you speak a little bit more about that? How O-GlcNAc affects susceptibility to disease.
>> Dr. Hanover: Yeah, so there's been several important studies; epidemiologic studies, the Dutch Hunger Winter study during World War II, in which the Dutch were starved, basically, as a population. And epidemiologic studies as follow-up, have shown that the offspring, particularly the grandchildren, of people who were subject to that environmental stress show increased levels of obesity, metabolic disease, and other deleterious—including cancer—other deleterious effects. This has been linked to DNA methylation, and that DNA methylation, we find, seems to be regulated by the O-GlcNAc pathway.
So, you can imagine you have a nutrient-sensing ligand modification very dependent on nutritional status that sits atop both DNA damage control and DNA methylation. And so we're excited by the possibility that this pathway, by integrating the stress response, particularly in the developing fetus and in their germ cells, in the fetus's own germ cells, could provide not only a means of conveying information to the fetus but also to their offspring. Really, truly transgenerational inheritance.
It's a very hard thing to study, but it's very important because a lot of metabolic disease has a genetic inheritance pattern but is modified by the environment. So, we're very interested in how these environmental factors that we study may increase the susceptibility to those genetic diseases which are driven by metabolic changes such as type 2 diabetes, Alzheimer's, and other chronic diseases.
>> Diego (interview): And can you describe the experiments you’re doing to study that? I’m assuming you’re starting with mice.
>> Dr. Hanover: Yes, currently we're studying that in the mouse where it's very straightforward thanks to recent technological developments to look at DNA methylation patterns, and we're able to do that genome-wide in the mouse. If you knock out the enzyme that removes O-GlcNAc, what you see is this remarkable decrease in total methylation, but more importantly, it's decrease in genes that subject to de novo methylation.
>> Diego (interview): De novo meaning something new instead of conserved from parent to offspring.
>> Dr. Hanover: Yeah, to something new. The O-GlcNAcase leads to destabilization of two of the key enzymes involved in de novo methylation. We think we understand the mechanism by which this is happening. The next step in this, of course, is to look at the primordial germ cells to see if that communication, if that environmental perturbation that we can induce genetically is translated into true changes in the primordial germ cells. If that's the case, then we have a really discrete and really the first example of how environmental information can be communicated to the genome. But we have a long way to go to prove it and certainly we have a long way to go to show this plays a key role in human biology, but the mouse studies are very promising.
>> Diego (interview): Wow, that’s seems like a big deal if you’re able to actually trace how the environment of the parent gets translated into the offspring. So what factors have you observed or found affect O-GlcNAc?
>> Dr. Hanover: Well, we know of a lot of things that will elevate O-GlcNAc. For example, high glucose. Gestational diabetes produces high glucose. We know that abrupt changes in temperature can affect O-GlcNAc transferase in this pathway. There's strong evidence that environmental toxicants can elevate O-GlcNAc. And all of these things, of course, are occurring in the inner uterine environment, affecting both the fetus and the placenta. We know there are huge changes in the placenta. We and others have examined that, but the next step is really to understand whether that stress is being conveyed across generations. And so when I say "stress," really, there are very few stressors that don't lead to changes in O-GlcNAc and that's why it's an appealing type model and hypothesis to suggest that it may be key to communicating that information to the genome.
>> Diego (interview): Got it. Are you looking at just the mother or can the father’s stress also affect the embryos genome?
>> Dr. Hanover: Yeah, so, you know, the primordial germ cell from both of the parents may be affected. And that was the interesting thing about the Dutch Hunger Winter study, is that they showed that the grandchildren of men exposed to the famine were the most dramatically affected. So, it seemed to be most strongly affecting methylation in the male germline. So, we're pursuing that both by looking at the male germline in mice and looking at primordial germ cells in both genders to look for changes in these imprinted regions of the genome.
>> Diego (interview): Makes sense. And how will you know if the “stress” gets transferred to the germline? Are you just looking at the cells or is the idea to look at the offspring and see if they share the same changes?
>> Dr. Hanover: Yes, yeah. The idea would be that we would do the environmental perturbation to the parents and then look for this subsequent changes in the offspring, but much as the epidemiologic studies that had been performed were done. I mean, nature performed this unfortunate experiment for us back in World War II, but we can recapitulate a lot of that in the lab using the mouse system.
>> Diego (interview): I see. Well shifting gears a little bit, because I always like to hear scientists’ origin stories. You started at NIH in Ira Pastan’s lab, who has been a guest on the podcast. What do you recall from those early days at NIH as a young scientist?
>> Dr. Hanover: Ah, excellent question. So, Ira's lab was an absolutely phenomenal place with huge levels of excitement. Those were the early days of oncogenes, so it was a really exciting time to be a young biologist. I guess all times are, frankly, but what I remember is that period was one where we now had the tools through direct cloning and sequencing to look at the molecular structure, at least at the primary sequence level of all these important molecules. So, it was sort of the first glimpse we had of the light at the end of the tunnel. We'd studied organellar biology and receptor biology for years, but now we actually had our hands on the primary sequence of these proteins, and so, you know, it really fueled a lot of things that led me to want to apply those guiding principles to glycobiology. At the time I started, there were very few glycobiologists doing that kind of work, trying to identify the molecules, the glycosyltransferases and the glycosidases. And so we were at a time when we could actually make a significant contribution just by doing routine cloning of these enzymes, and that was a very exciting time.
>> Diego (interview): Yeah, I bet. Well, you only grew your career from there and became a PI in the intramural research program. So, any advice for junior scientists already in the IRP who want to do the same?
>> Dr. Hanover: I guess the intramural program has some real advantages. We don't really work on four to five-year funding cycles where you're evaluated on what you've done the previous four years, and more importantly, what you propose to do. In the intramural program, you can take risks that are really probably unheard of in the extramural environment. I was able to study the O-GlcNAc pathway, even though I was given advice from all my colleagues that this was not something that was going to be fruitful, but I just decided that we didn't understand it very well and it was going to be important, question is how. So, persistence paid off in that we were among the very first few people to study this modification, and I think that's generally true, that we're in an environment here where we can take some risks and those risks are worth taking, particularly for young people. I don’t want to leave the impression that it was easy. We're under scrutiny, make no mistake about it. We have to be productive. We have to make advances. But the kinds of advances that we make don't have to be limited to what we propose on paper. Now, I don't want to leave the impression that people in extramural environment only work on what their grant suggests, but it does limit their freedom to take real chances. In my case, to work on model systems that we had never worked on, C. elegans in my case, and I'd worked on the fly before, but these model systems really came in handy in trying to understand this modification. So, my advice would be take some risks, and my lab chief, Bill Jacoby, who when I first started as an independent investigator gave me that same advice, he said, "This is the one place where you can do this stuff. Do what you want to do."
>> Diego (interview): Mhm, well I can’t think of better way to close out the episode. So, thank you so much and thank you for your time, Dr. Hanover.
>> Dr. Hanover: No, I appreciate it. That was fun.
Related Episodes
This page was last updated on Monday, September 30, 2024