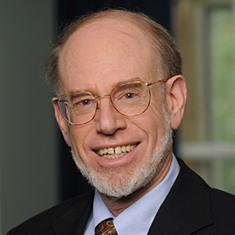
Research Topics
T Lymphocyte Recognition of Antigens and Applications to Vaccines for AIDS and Cancer
Our research focuses on elucidating new fundamental principles governing T cell activation, regulation, and effector function, and employing these to develop more effective vaccine and immunotherapy strategies for HIV, cancer, and viruses causing cancer.
This involves several steps that together comprise a push-pull approach. First, we optimize the antigen to improve immunogenicity by epitope enhancement, changing the amino acid sequence to increase affinity for the relevant major histocompatibility (MHC) molecule. We have done this for several cancer antigens, including the prostate cancer antigen, TARP, and have now completed a phase I/II clinical trial in Stage D0 prostate cancer patients with rising PSA levels to determine whether the TARP peptide vaccine can reduce the rate of PSA rise. The slope of PSA rise was significantly reduced in 74% of patients at one year. A randomized controlled phase II trial is open and accruing patients.
The second step is to push the response with molecular adjuvants, such as cytokines and Toll-like receptor (TLR) ligands, to improve not only the quantity but also the quality of the response (which we have found often to be more important). We published that IL-15 is an important mediator of CD4 T cell help for CD8 T cells, in that it is sufficient to substitute for help in animals depleted of CD4 T cells, to allow a memory CD8 response and prevent TRAIL-mediated apoptosis, and also it is necessary for help. We also found that IL-15 increased the avidity of the CD8 T cells, necessary for effective clearance of virus or tumor cells. We extended this research to human CD8 T cells and showed that a primary in vitro CD8 T cell response was dependent on CD4 help but that IL-15 could be substituted, but not IL-2. IL-15 also restored the in vitro responses of CD8 T cells from HIV-infected individuals. We also found that IL-15 affects DC and CD4+ T cell subpopulations in the gut mucosa. We recently found that IL-21 is necessary to optimize activation of interferon-stimulated genes (ISGs) in gut CD4 T cells to effectively clear a Citrobacter rodentium colitis. This has implications for vaccines as well as inflammation in the gut.
We also investigated TLR ligands as adjuvants, because these can mature DCs and induce their production of cytokines like IL-12 and IL-15. We published a study showing synergy between pairs of TLR ligands that work through different intracellular signal transducers, MyD88 or TRIF, and determined the mechanism in DCs involving unidirectional cross-talk from TRIF to enhance MyD88-dependent cytokine production. We found a triple TLR ligand combination that induces more effective protection against virus infection. This combination does not increase T cell quantity, but improves quality by inducing higher avidity T cells, and induces more IL-15 production, accounting for the higher avidity. We tested the triple TLR ligands, IL-15, both or neither as vaccine adjuvants in a peptide-prime, MVA-boost mucosal vaccine for SIV in macaques, challenging intrarectally with SIVmac251. Only the macaques receiving both types of adjuvants showed some protection, so we investigated correlates of protection. In the adaptive immune arm, surprisingly only polyfunctional CD8 T cells specific for SIV antigens, but not total specific T cells measured by peptide-MHC tetramer binding, correlated with protection. In the innate immune arm, we found the adjuvants induced long-lived innate protection induced by the adjuvant combination. In subsequent studies, we found that vaccine protection against SHIV or SIV acquisition intrarectally can occur in the absence of anti-envelope antibodies, contrary to expectation, and may depend on a combination of T cells and trained innate immunity involving monocyte memory. We also found that some vaccine components can induce regulatory cells (see below). Thus, vaccine strategies that induce both innate and adaptive immunity but avoid inducing immunosuppressive cells may be the most efficacious.
The third step is to target the immune response to the relevant tissue, the mucosa in the case of HIV. We have previously compared mucosal versus subcutaneous delivery of a peptide AIDS vaccine in macaques and found that only the mucosal route induced CD8 T cells in the gut mucosa and was more effective at reducing virus load even in the blood because it more effectively cleared virus from the major reservoir for SIV replication in the gut mucosa that was seeding the bloodstream. We further found that induction of such CD8 T cells in the gut mucosa before intrarectal challenge with an AIDS virus could significantly delay dissemination of virus from the initial site of infection in the mucosa to the bloodstream. We conclude that the local CD8 T cell response was able to reduce the initial nidus of infection at the site of transmission, and that if we could more completely eradicate the virus at the initial nidus of infection, we might nip it in the bud and abort the infection before it disseminated and became established. We showed that this protective effect was correlated with induction of high avidity CD8 T cells in the gut mucosa, and not with CD8 T cells in the blood or peripheral lymph nodes. We also published a new paradigm for induction of CD8 T cell immunity directly in vaginal mucosa (a type II mucosa that lacks organized lymphoid structures), independent of draining lymph nodes or other lymphoid structures, overturning textbook dogma.
Development of a mucosal vaccine for HIV is most important because 85-90% of transmission is through a mucosal route, either genital or gastrointestinal, and because the major site of replication is in the gut mucosa, regardless of the route of transmission. We then devised a nanoparticle vaccine coated in a way to allow release in either the small or large intestine after oral delivery. Delivery to the large intestine mimicked intrarectal immunization by a more practical route, and achieved protective T and B cell immunity against both rectal and vaginal viral challenge, whereas delivery to the small intestine did not. We have translated these findings to non-human primate studies where we have evidence of protection in two independent macaque vaccine studies, as the preclinical basis for a human clinical trial. The protection could occur in the absence of anti-envelope antibodies, implying that either T cell immunity or trained innate immunity played the critical role. Indeed, we now have data confirming induction of trained innate immunity in monocytes of vaccinated macaques, correlating with protection. In the process, we discovered that the small and large intestinal mucosae are separate subcompartments in which we can selectively induce a response by targeting the antigen for release in one or the other. This implies different homing receptors for T cells to each compartment. We found that colonic DCs program T cells to preferentially return to the colon, in contrast to small intestinal DCs that induce homing to the small intestine, attributable to different levels of retinoic acid and induction of different chemokine receptors. We also found that colonic DCs can patrol the gut lumen near colon patches under chemokine control and bring antigen back to the lamina propria.
The fourth step is to pull the response by removing the brakes, i.e., blocking the negative regulatory mechanisms that inhibit the immune response. We previously discovered a new immunoregulatory pathway involving NKT cells that suppresses tumor immunity. The NKT cells make IL-13 that induces myeloid cells to make TGF-β, which suppresses the CD8 T cell response. However, as we and others have also seen that NKT cells can protect against tumors, we needed to resolve this paradox. We found that type I NKT cells (using an invariant TCRα chain) protected, whereas type II NKT cells (using diverse T cell receptors) suppressed immunity. Moreover, selective activation of type I or type II NKT cells showed they cross-regulated each other, defining a new immunoregulatory axis, analogous to the axis between Th1 and Th2 cells that has profoundly affected immunology. The NKT cells are among the first responders, so the balance along the NKT axis could influence subsequent adaptive immune responses. We found that type II NKT cells also suppress conventional CD4 and CD8 antigen-specific T cells, so they are broadly suppressive. We are examining the mechanisms of suppression and also investigating the relationship between suppressive NKT cells and CD25+ Foxp3+ T regulatory cells.
In one tumor model we find that both Treg cells and type II NKT cells can suppress tumor immunity, but that the effect of the latter is counterbalanced by type I NKT cells, so this third T cell regulates the balance between the regulators. In trying to tip the balance along the NKT regulatory axis, we find that blocking IL-13 can delay growth of spontaneous, autochthonous tumors even in HER-2 transgenic mice that develop aggressive independent tumors in all 10 mammary glands. Conversely, stimulating with a type I NKT cell agonist can protect against tumors. We also found that the type of immunoregulation that dominates depends on the tissue location, even of the same tumor. In the lungs, type II NKT cells regulated growth of CT26 tumors whereas in the skin, Treg cells dominated, and there was no crosstalk between the sites. When the suppressors were removed, the unmasked effectors cross-protected in only one direction, from skin to lung. Thus, a primary tumor and its metastases in a different tissue may require different types of immunotherapy, and this needs to be taken into account in clinical practice.
We have been characterizing type II NKT cells and studying ways to activate or inhibit them. A major subset of these recognize sulfatide presented by CD1d, and we have been able to stain them with sulfatide-CD1d tetramers These cells are most prevalent in the lung and the liver, two tissues that are also common sites of tumor metastases. We are studying their role in metastasis. We are also performing structure-function studies with sulfatide analogues to either stimulate or inhibit these immunoregulatory NKT cells.
We discovered a new class of NKT cell agonist represented by beta-mannosylceramide, that protects against tumors by a novel mechanism dependent on TNF and nitric oxide synthase (NOS) but not on interferon-gamma, distinct from that of alpha-galactosylceramide which is dependent on interferon-gamma and not on TNF or NOS. We are exploring the mechanism of this protection, but because this new agonist avoids natural alpha-gal antibodies and induces less anergy than alpha-GalCer, and because it synergizes with alpha-GalCer and also stimulates human iNKT cells, we are pursuing it as an immunomodulator to improve immunity against human cancer. We are comparing different NKT agonists, so that we may be able to tailor the protective response to fit different conditions and to achieve synergy between different mechanisms of protection. We also examined an intratumoral chemotherapy with an uptake enhancer, INT230-6, that converts an established tumor in mice into an endogenous cancer vaccine that induces T cell immunity and memory that is necessary for eradication of the tumor, and that synergizes with checkpoint inhibitors.
A key mediator of the NKT regulatory pathway and an important regulator of T regulatory cells is TGF-β. We have found that blockade of TGF-β can protect against certain tumors in mice, and can synergize with anti-cancer vaccines in two mouse models. The protection is dependent on CD8 T cells, and when used in combination with a vaccine, the anti-TGF-β increases the number of both total and high avidity CD8 T cells. We have translated this into a human clinical trial of a human anti-TGF-β monoclonal antibody in a CRADA with Genzyme, mostly in melanoma. The phase I study showed some activity including one 89% partial remission and several cases of mixed responses or stable disease. We also found that it is sufficient to block only TGF-beta 1 and 2, without blocking isoform 3, to achieve protection in mouse cancers or synergize with mouse cancer vaccines. The combination of cancer vaccine and anti-TGF-beta can be further improved by adding anti-PD-1, and the triple combination is more effective than any pairwise combination. We would like to combine anti-TGF-beta with anti-PD-1 and our cancer vaccines to more effectively induce anti-cancer immunity in human cancer patients.
Another important mediator of negative regulation is the myeloid-derived suppressor cell (MDSC). This population is known to increase in cancer and HIV infection. We have now found that it can also be increased by vaccines, and can thus reduce the efficacy of the vaccine. We found that rhesus macaques given an AIDS vaccine intrarectally had more MDSCs than animals given the same adjuvants without the vaccine, and that protection was diminished by the presence of the MDSCs. T cell activity was increased by depletion of MDSCs, and the MDSCs were shown to suppress when added into T cell cultures. Thus, vaccine design must take into account ways to prevent this vaccine-induction of MDSCs. We also found that SIV infection can affect the trafficking and survival of MDSCs in macaques, in part because we showed these cells could surprisingly be infected with SIV.
Finally, we published that an adenovirus vaccine expressing the extracellular and transmembrane domains of HER-2 can cure large established mammary cancers and lung metastases in mice. The mechanism surprisingly involves antibodies that inhibit HER-2 function, rather than T cells. We have now made a similar recombinant adenovirus expressing the human HER-2 domains and are completing a clinical trial in human cancer patients with metastatic cancers who progressed on standard therapies but who are naive to HER2-targeted therapies, and have recently opened a second part to treat patients who have progressed on previous HER2-directed therapies. The vaccine consists of autologous DCs transduced with the Ad-HER2 vector. So far, although no responses were seen at the lowest dose level in Part 1, of 10 evaluable patients at the second and third dose levels, 5 had either CR, PR, or stable disease, indicating clinical benefit. We encourage referrals for both this HER2 vaccine trial and the TARP prostate cancer vaccine trial described above.
Biography
Dr. Jay A. Berzofsky was appointed Chief, Vaccine Branch, Center for Cancer Research, National Cancer Institute, in 2003, after being Chief, Molecular Immunogenetics and Vaccine Research Section, since 1987. He graduated Summa cum Laude from Harvard (1967), and received a Ph.D.-M.D. from Albert Einstein College of Medicine (AECOM). After interning at Massachusetts General Hospital, he joined NIH in 1974. Dr. Berzofsky's research has focused on antigen processing/presentation, epitope structure, cytokine and regulatory cell control of T cell function and avidity, NKT cells, and translation to the design and clinical trials of vaccines for AIDS, cancer, and viruses causing cancer. He has 521 scientific publications. He was elected President of the American Society for Clinical Investigation (1993-94), a member of the Association of American Physicians, a Fellow of the American Association for the Advancement of Science (AAAS), and Distinguished Alumnus of the Year for 2007 by AECOM. He was elected Chair of the Medical Sciences Section of the AAAS for 2007-2008. He received the NIH Director’s Award and the NCI Merit Award in 2008, another NCI Director’s Merit Award in 2011, and a Career Award “for his important contribution to tumor immunotherapy” from the European Academy of Tumor Immunology in 2018.
Selected Publications
- Wood LV, Fojo A, Roberson BD, Hughes MS, Dahut W, Gulley JL, Madan RA, Arlen PM, Sabatino M, Stroncek DF, Castiello L, Trepel JB, Lee MJ, Parnes HL, Steinberg SM, Terabe M, Wilkerson J, Pastan I, Berzofsky JA. TARP vaccination is associated with slowing in PSA velocity and decreasing tumor growth rates in patients with Stage D0 prostate cancer. Oncoimmunology. 2016;5(8):e1197459.
- Zhu Q, Talton J, Zhang G, Cunningham T, Wang Z, Waters RC, Kirk J, Eppler B, Klinman DM, Sui Y, Gagnon S, Belyakov IM, Mumper RJ, Berzofsky JA. Large intestine-targeted, nanoparticle-releasing oral vaccine to control genitorectal viral infection. Nat Med. 2012;18(8):1291-6.
- Terabe M, Ambrosino E, Takaku S, O'Konek JJ, Venzon D, Lonning S, McPherson JM, Berzofsky JA. Synergistic enhancement of CD8+ T cell-mediated tumor vaccine efficacy by an anti-transforming growth factor-beta monoclonal antibody. Clin Cancer Res. 2009;15(21):6560-9.
- Terabe M, Swann J, Ambrosino E, Sinha P, Takaku S, Hayakawa Y, Godfrey DI, Ostrand-Rosenberg S, Smyth MJ, Berzofsky JA. A nonclassical non-Valpha14Jalpha18 CD1d-restricted (type II) NKT cell is sufficient for down-regulation of tumor immunosurveillance. J Exp Med. 2005;202(12):1627-33.
- Terabe M, Matsui S, Noben-Trauth N, Chen H, Watson C, Donaldson DD, Carbone DP, Paul WE, Berzofsky JA. NKT cell-mediated repression of tumor immunosurveillance by IL-13 and the IL-4R-STAT6 pathway. Nat Immunol. 2000;1(6):515-20.
Related Scientific Focus Areas
Microbiology and Infectious Diseases
View additional Principal Investigators in Microbiology and Infectious Diseases
This page was last updated on Tuesday, June 25, 2024