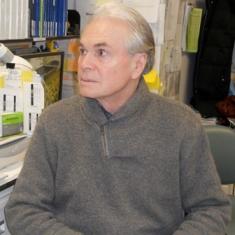
Research Topics
Our overall goal is to understand why particular chromosomal regions and genes are prone to generate genomic disorders. Relevant specialized features of the human genome, including some that are especially relevant to cancer, have increasingly become the focus of our research. Involved are: 1) aspects of gene duplication and evolution and 2) specialized features of mitosis -- particularly the centromere structure and function that underlie proper chromosome segregation. The first of these topics, gene duplication and evolution, is the basis for divergence and in some cases for the supply of variants of genes that predispose to cancer. The second topic, centromere structure and function, is directly involved in processes that, when aberrant, lead to aneuploidy and polyploidy, which are hallmarks of many cancers.
Topic 1: Gene Duplication, Evolution, Diversifying Selection, and Cancer
Diversifying selection, which accelerates genetic variation, affects a subset of genes, most of which are involved in host defense and sexual reproduction. However, recent studies suggest that diversifying selection may also act on tumor suppressor genes. Because most tumors develop in organisms that are well past their reproductive prime, the tumor phenotype is not likely to be subject to natural selection. Thus, selective pressures on tumor suppressor or tumor-promoting genes most likely relate to another physiological role, possibly in early development or another life stage of the organism. The hypothesis of "developmental evolution" suggests that multifunctional genes that play a role in adaptive evolution are also associated with disease predisposition.
To identify new genes subject to diversifying selection, we reconstructed an evolutionary history of six loci: BRCA1, which is linked to breast cancer susceptibility; ASPM, which is linked to microcephaly; ATM, which is responsible for the human genetic disorder ataxia telangiectasia; NBS1, which is responsible for Nijmegen breakage syndrome; DRD3, which is the dopamine D2-like receptor gene; and the HPCX locus/SPANX gene cluster, which is linked to prostate cancer susceptibility. Syntenic regions of these loci were isolated by recombinational TAR cloning from a representative group of nonhuman primates.
Analysis of these data showed that three genes, ASPM, BRCA1, and SPANX have been subject to diversifying selection in recent history. Interspecies protein sequence comparisons provided evidence of accelerated evolution at ASPM, possibly prior to brain size expansion in hominids. Because positive selection acts only when the fitness of the organism increases or decreases, these data are consistent with the hypothesis that ASPM is a key determinant of brain size in hominids. Our recent studies also showed that ASPM is expressed in all adult tissues, and that it is up-regulated in a wide variety of cancers. Given the rapid evolution of ASPM, additional studies are needed to clarify its role in carcinogenesis.
Our recent discovery of the amplification of the SPANX gene family at the Hereditary Prostate Cancer on chromosome X (HPCX) locus suggests that these genes could still be evolving in humans. It is intriguing that SPANX genes reside within 20-100 kb segmental chromosomal duplications (SDs) with a high level of sequence similarity. It is well documented that SDs mediate ectopic interactions leading to chromosomal rearrangements such as duplications, deletions, and inversions. These observations suggest that the predisposition to prostate cancer in some HPCX families may have resulted from genomic rearrangements mediated by SDs. Additional studies are in progress to characterize such rearrangements in the SPANX gene cluster.
Topic 2: Centromere Structure and Function
Centromeric regions of mammalian chromosomes exhibit a high rate of evolutionary change. Despite their importance for proper chromosome segregation, the structure and function of human centromeres remain relatively poorly understood. This is at least in part because of the highly repetitive nature of centromeric DNA, which has made it difficult to clone, sequence and study. However, two independent groups recently reconstituted a functional human centromere using centromere-derived alphoid DNA arrays. This accomplishment has made it possible to construct human artificial chromosomes (HACs) and to begin to analyze the structural requirements for de novo kinetochore formation.
Earlier studies suggested that higher-order repeat alphoid DNAs (HORs) containing CENP-B boxes (17 bp binding sites for CENP-B) were sufficient for HAC formation, but technical issues interfered with more detailed studies of the structural requirements for centromere function. To address these needs, a new strategy was developed in our section to manipulate alphoid DNA arrays using RCA-TAR. This approach involves two steps: rolling circle amplification (RCA) of an alphoid DNA dimer and assembly of the amplified fragments by transformation-associated recombination in yeast (TAR). Because the alphoid DNA sequence can be manipulated before the amplification step, it is possible to introduce mutations, insert recognition sites for DNA-binding proteins, or otherwise vary the alphoid DNA sequence and/or structure. Using this system, synthetic alphoid DNA arrays from 30 to 140 kb have been generated and characterized. This work provided the first information on the minimal alphoid DNA sequence required to seed a functional kinetochore.
Recently, we exploited the RCA-TAR technique in order to develop a new generation of HACs with a conditional centromere. A synthetic alphoid DNA array in these HACs resembles normal centromeric repeats, but contains a tetracycline operator sequence in every second alphoid monomer. This allows the targeting of a wide range of proteins into the functional centromere as fusions to tetracycline repressor; some of them disrupt kinetochore structure and function. These HACs are now being used to investigate the protein interactions within the kinetochore and to define the epigenetic signature of centromeric chromatin. They may also be useful for gene therapy research and for other applications.
Collaborators on this research are Victor Lobanenkov and Arya Biragyn, NIH; William Earnshaw, University of Edinburgh, UK; Johanna Schleutker, Institute of Medical Technology, University of Tampere, Finland; Hiroshi Masumoto, Nagoya University, Japan; and Mitsuo Oshimura, Tottori University, Japan.
Biography
Selected Publications
- Kim JH, Kononenko A, Erliandri I, Kim TA, Nakano M, Iida Y, Barrett JC, Oshimura M, Masumoto H, Earnshaw WC, Larionov V, Kouprina N. Human artificial chromosome (HAC) vector with a conditional centromere for correction of genetic deficiencies in human cells. Proc Natl Acad Sci U S A. 2011;108(50):20048-53.
- Kim JH, Ebersole T, Kouprina N, Noskov VN, Ohzeki J, Masumoto H, Mravinac B, Sullivan BA, Pavlicek A, Dovat S, Pack SD, Kwon YW, Flanagan PT, Loukinov D, Lobanenkov V, Larionov V. Human gamma-satellite DNA maintains open chromatin structure and protects a transgene from epigenetic silencing. Genome Res. 2009;19(4):533-44.
- Kouprina N, Mullokandov M, Rogozin IB, Collins NK, Solomon G, Otstot J, Risinger JI, Koonin EV, Barrett JC, Larionov V. The SPANX gene family of cancer/testis-specific antigens: rapid evolution and amplification in African great apes and hominids. Proc Natl Acad Sci U S A. 2004;101(9):3077-82.
- Kouprina N, Pavlicek A, Mochida GH, Solomon G, Gersch W, Yoon YH, Collura R, Ruvolo M, Barrett JC, Woods CG, Walsh CA, Jurka J, Larionov V. Accelerated evolution of the ASPM gene controlling brain size begins prior to human brain expansion. PLoS Biol. 2004;2(5):E126.
- Larionov V, Kouprina N, Graves J, Chen XN, Korenberg JR, Resnick MA. Specific cloning of human DNA as yeast artificial chromosomes by transformation-associated recombination. Proc Natl Acad Sci U S A. 1996;93(1):491-6.
Related Scientific Focus Areas
Molecular Biology and Biochemistry
View additional Principal Investigators in Molecular Biology and Biochemistry
This page was last updated on Wednesday, November 8, 2023