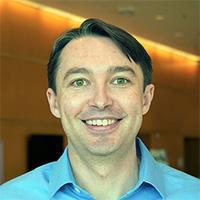
J. Robert Hogg, Ph.D.
Senior Investigator
Ribonucleoprotein Biochemistry
NHLBI
Research Topics
The process of gene expression regulation depends on timely synthesis and degradation of messenger RNAs (mRNAs). The goal of the Laboratory of Ribonucleoprotein Biochemistry is to understand how cells build RNA-protein complexes so they may destroy mRNAs at the right times and places. Within this overarching goal, the laboratory focuses on two major areas: 1) the biochemical and biophysical processes responsible for achieving specific RNA decay and 2) the cellular consequences of altered decay.
The laboratory primarily studies these questions in the context of nonsense-mediated mRNA decay (NMD), an evolutionarily conserved pathway that performs important quality control and regulatory functions. As a quality control pathway, NMD degrades mRNAs that contain premature termination codons, a class of gene alteration responsible for ~15% of human genetic disease alleles. Further, the NMD pathway plays a major role in gene expression regulation, impacting as many as 10% of all human genes.
We use experimental systems ranging from in vitro biochemistry to whole-transcriptome studies of RNA expression and RNA-protein interactions. The resources of the Intramural Research Program allow us to be flexible in our approach to understanding NMD and other post-transcriptional regulatory mechanisms.
How do cells balance specificity and efficiency in NMD regulation?
Many human mRNAs contain long 3’ untranslated regions (3’UTRs), a feature that can make them susceptible to NMD. However, only a subset of human mRNAs with long 3’UTRs are degraded by the pathway. Over several papers, we have developed evidence that a major mechanism for NMD-resistance of transcripts is recruitment of specific RNA-binding proteins to the vicinity of stop codons. These RNA-binding proteins, exemplified by PTBP1 and hnRNP L, mark genuine stop codons to prevent their recognition by the NMD pathway. Unexpectedly, we have also found that this protective mechanism allows expression of aberrant BCL2 transcripts that promote cell survival in B cell lymphomas.
UPF1 is a highly nonspecific RNA binding protein, raising the question of how sequence-specific RNA-binding proteins such as PTBP1 and hnRNP L can effectively suppress UPF1 binding to long 3’UTRs. Using in vitro, ex vivo, and cellular assays, we have uncovered a novel mechanism in which PTBP1 induces UPF1 to dissociate from RNA when UPF1 hydrolyzes ATP. This mechanism relies on a short regulatory loop in the helicase core of UPF1, and we have shown that a naturally occurring alternative UPF1 isoform containing an extension of this loop (termed long-loop UPF1 or UPF1LL) is able to overcome the protective proteins.
How does the NMD pathway respond to changes in cellular conditions?
The NMD pathway depends on translation to identify and degrade target mRNAs. Studies from many labs have shown that inhibition of translation greatly reduces decay of canonical NMD target mRNAs. However, under stress and other physiological conditions that inhibit cap-dependent translation, translational activity is not completely abolished. This means that translation termination events potentially susceptible to NMD persist when translation is partially inhibited by stress or pharmacological agents. By looking for conditional NMD targets, we have found that the specificity of the NMD pathway is dramatically remodeled under conditions of partial translational repression. The shift in NMD specificity in response to changes in translation is driven by the alternative UPF1LL isoform, which maintains activity under conditions that inhibit of the major UPF1 isoform. In this way, the UPF1LL isoform allows cells to re-program NMD to adjust to changing cellular conditions.
How do the biochemical and biophysical properties of the UPF1 helicase contribute to proper NMD execution?
UPF1 is an RNA helicase, meaning that it hydrolyzes ATP to perform mechanical work, including unwinding double-stranded RNA, translocating on single-stranded RNA, and disrupting RNA-protein complexes. While UPF1 proteins lacking ATPase activity are unable to properly conduct NMD, the importance of UPF1 helicase and translocase activities remains unclear. Working with NHLBI biophysicist Keir Neuman, we are investigating how the biochemical and biophysical properties of UPF1 influence NMD efficiency and specificity in cells. We have discovered that UPF1 mutants with greatly impaired helicase and translocase activities are nevertheless able to conduct efficient NMD. Together with our work on the UPF1 “long-loop” isoform, our findings point to a model in which ATPase-stimulated dissociation of UPF1, rather than UPF1 helicase activity per se, is important for maintaining cellular NMD function.
How do cells exploit NMD for post-transcriptional regulation of gene expression?
NMD is best known as a quality control mechanism, but the pathway performs important gene expression regulatory functions in all eukaryotes studied to date. The regulatory substrates of NMD include genes containing ultraconserved elements, stretches of 200 or more base pairs that are identical among humans, mice, and rats. Several important proteins involved in pre-mRNAs splicing are controlled via alternative splicing and NMD of UCEs. We have investigated the interactions and function of a largely overlooked UCE-containing gene, the human zinc finger RNA-binding protein (ZFR). We have identified ZFR as a major regulator of cassette and mutually exclusive exon splicing, with a particularly important role in suppressing innate immune responses. Using UV crosslinking and high-throughput sequencing, we have discovered that ZFR binds long stretches of target introns to promote the inclusion of cassette exons. This unusual mode of binding and the involvement of ZFR in numerous important alternative splicing events raise many questions about mechanisms of alternative splicing regulation and the role of non-canonical splicing factors in gene expression regulation.
How has the NMD pathway evolved in response to the enormous complexity of the mammalian transcriptome
The NMD pathway is conserved throughout eukaryotes, from single-celled fungi to humans. With increasing organismal complexity comes increasing transcriptome complexity, driven by alternative pre-mRNA processing. To effectively perform quality control and gene expression regulatory roles, the NMD machinery has correspondingly evolved to include new proteins. In collaboration with the laboratory of James Inglese (National Center for Advancing Translational Science), we have used whole-genome siRNA screening to discover new human genes required for efficient NMD. These new players in NMD include the ICE1 protein, which interacts with the exon junction complex to promote the link between pre-mRNA splicing in the nucleus and translation-dependent decay in the cytoplasm. We are now working to understand the biochemical and cellular functions of ICE1 and other proteins identified in our screen. One area of particular interest is understanding how metazoan-specific NMD factors determine cell-type and condition-specific regulation of RNA processing and decay.
Tools for studying mRNP assembly, translation, and RNA decay
mRNAs are made, translated, and destroyed in the context of large ribonucleoprotein complexes (RNPs). These complexes are compositionally heterogenous and dynamic, presenting a major challenge to understanding their biogenesis and function. We have developed tools that allow us to purify endogenously assembled mRNPs for proteomic or biochemical studies. Using the coat protein from the Pseudomonas phage 7 (PP7), we can purify tagged RNAs and associated proteins from cultured cells and comprehensively identify protein components of mRNPs by tandem mass spectrometry. Recently, we have shown that affinity purified mRNPs can be highly efficiently translated in vitro under physiological buffer conditions and have developed methods to study protein dissociation from mRNPs immobilized on magnetic beads. These tools provide a generalizable platform for investigating mRNP composition and function.
Biography
J. Robert Hogg graduated from Haverford College in 2000 with a B.S. in biology and earned a Ph.D. from the Department of Molecular and Cell Biology at the University of California, Berkeley in 2006. His thesis research at UC Berkeley focused on the composition and function of ribonucleoprotein complexes containing noncoding RNAs. He pursued postdoctoral research at Columbia University, where he studied mechanisms of mRNA biogenesis and quality control. He joined the NHLBI in 2012 as an Earl Stadtman tenure-track Investigator.
Selected Publications
- Fritz SE, Ranganathan S, Wang CD, Hogg JR. An alternative UPF1 isoform drives conditional remodeling of nonsense-mediated mRNA decay. (external link) EMBO J. 2022;41(10):e108898.
- Kishor A, Ge Z, Hogg JR. hnRNP L-dependent protection of normal mRNAs from NMD subverts quality control in B cell lymphoma. (external link) EMBO J. 2019;38(3).
- Baird TD, Cheng KC, Chen YC, Buehler E, Martin SE, Inglese J, Hogg JR. ICE1 promotes the link between splicing and nonsense-mediated mRNA decay. (external link) Elife. 2018;7.
- Haque N, Ouda R, Chen C, Ozato K, Hogg JR. ZFR coordinates crosstalk between RNA decay and transcription in innate immunity. (external link) Nat Commun. 2018;9(1):1145.
- Fritz SE, Ranganathan S, Wang CD, Hogg JR. The RNA-binding protein PTBP1 promotes ATPase-dependent dissociation of the RNA helicase UPF1 to protect transcripts from nonsense-mediated mRNA decay. (external link) J Biol Chem. 2020;295(33):11613-11625.
Related Scientific Focus Areas
Molecular Biology and Biochemistry
View additional Principal Investigators in Molecular Biology and Biochemistry
This page was last updated on Tuesday, June 10, 2025