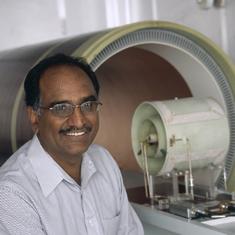
Research Topics
Magnetic Resonance Imaging of Tumor Physiology
Characterizing tumors and related pathological conditions on a physiologic basis by noninvasive radiological techniques is a potentially useful capability for diagnosis as well as for devising appropriate cancer treatment approaches. Magnetic resonance imaging (MRI) is a commonly used clinical imaging procedure that provides images of soft tissue anatomy with excellent detail. Recent advances have made it possible to provide functional information such as brain function using functional MRI (fMRI) techniques. To obtain physiological information such as oxygen or tissue redox stats in pathological situations such as solid tumors or ischemic/hypoxic tissue, quantitatively and noninvasively, we are developing imaging techniques based on electron paramagnetic resonance (EPR). EPR is similar to nuclear magnetic resonance (NMR) in many ways. NMR probes nuclei such as 1H, 13C, and 31P, while EPR detects paramagnetic species. Paramagnetic species by definition contain unpaired orbital electrons. Examples of paramagnetic species are free radicals and transition metal complexes such as iron and copper. While EPR spectroscopy is used to detect metal complexes and free radicals for medical imaging applications, stable free radicals with simple EPR spectra are desirable. Examples of free radicals, which can be used at nontoxic levels for in vivo imaging, are nitroxides and trityl radicals. These species have simple EPR spectra and have long in vivo pharmacological half-lives. The intrinsic EPR spectral property of the free radical, such as the spectral bandwidth (full width of the absorption band at half maximum) is directly related to oxygen concentration. Imaging modalities, which can map the spatial distribution of the free radical concentration and extract the spectral information on a pixel-by-pixel basis, will therefore provide oxygen maps of the object being imaged. Based on this theme, we have developed instrumentation for in vivo detection and imaging of free radical contrast agents that can be detected and imaged in experimental animals such as mice and rats.
Three separate imaging configurations for the detection and imaging of free radicals are being developed and evaluated for medical noninvasive imaging applications:
- FT EPR imaging. With this method, the free radicals are imaged by collecting their responses after pulsed excitation under static gradient magnetic fields. Using this technique, we are able to obtain pO2 images within 2 minutes.
- Continuous wave EPR imaging. Using free radical contrast agents, which participate in redox reactions in tissue, it is possible to perform pharmacokinetic imaging experiments in which the free radical tracer is converted to a nonmagnetic species by intracellular redox processes. Using this imaging technique, it will be possible to examine differences in redox status between normal and pathological conditions noninvasively.
- Overhauser enhanced MRI (OMRI). This is a hybrid technique, which uses the inherent sensitivity of EPR spectroscopy to enhance the intensity of images obtained by conventional MRI. This technique therefore requires a free radical contrast agent like in EPR imaging. The object to be imaged is infused with the free radical contrast agent and placed in a coil, which is tuned to the resonant frequency of the electron and proton. The MRI sequence is preceded by RF irradiation specific to the free radical. The extent of image enhancement is inversely dependent on the concentration of oxygen. Using this technique, it is possible to obtain anatomically coregistered pO2 maps in less than 10 minutes. Our studies in tumor-bearing mice show that tumors of ~1 cm in diameter contain regions of significantly hypoxic regions as well as normoxic regions.
We are assessing these three techniques as potential functional imaging modalities, which can be hopefully used in humans to provide important physiologic information.
Biography
Dr. Krishna obtained his Ph.D. in physics from the Indian Institute of Technology, Madras, India, in 1984 and joined the NCI the same year. He became an independent investigator in 1993. His research interests include studies of free radical processes in causing oxidative injury and antioxidant defense. More recently, he has been involved in developing functional imaging tools to study tumor physiology.
Selected Publications
- Matsumoto S, Saito K, Takakusagi Y, Matsuo M, Munasinghe JP, Morris HD, Lizak MJ, Merkle H, Yasukawa K, Devasahayam N, Suburamanian S, Mitchell JB, Krishna MC. In vivo imaging of tumor physiological, metabolic, and redox changes in response to the anti-angiogenic agent sunitinib: longitudinal assessment to identify transient vascular renormalization. Antioxid Redox Signal. 2014;21(8):1145-55.
- Li F, Pang X, Krausz KW, Jiang C, Chen C, Cook JA, Krishna MC, Mitchell JB, Gonzalez FJ, Patterson AD. Stable isotope- and mass spectrometry-based metabolomics as tools in drug metabolism: a study expanding tempol pharmacology. J Proteome Res. 2013;12(3):1369-76.
- Enomoto A, Hirata H, Matsumoto S, Saito K, Subramanian S, Krishna MC, Devasahayam N. Four-channel surface coil array for 300-MHz pulsed EPR imaging: proof-of-concept experiments. Magn Reson Med. 2014;71(2):853-8.
- Krishna MC, Matsumoto S, Saito K, Matsuo M, Mitchell JB, Ardenkjaer-Larsen JH. Magnetic resonance imaging of tumor oxygenation and metabolic profile. Acta Oncol. 2013;52(7):1248-56.
- Jang H, Subramanian S, Devasahayam N, Saito K, Matsumoto S, Krishna MC, McMillan AB. Single acquisition quantitative single-point electron paramagnetic resonance imaging. Magn Reson Med. 2013;70(4):1173-81.
Related Scientific Focus Areas
Biomedical Engineering and Biophysics
View additional Principal Investigators in Biomedical Engineering and Biophysics
This page was last updated on Thursday, June 5, 2025