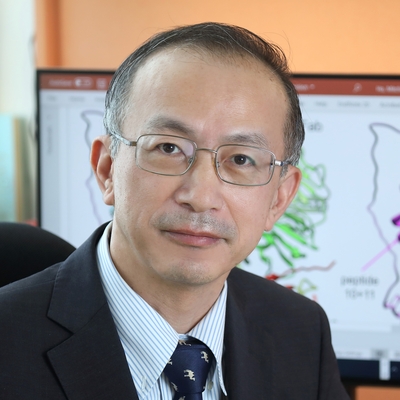
Research Topics
Dr. Ho's laboratory conducts research in biochemistry and molecular biology, with a focus on glypican biology, antibody protein engineering, and cancer immunology. With a primary interest in the mechanisms of cell surface receptors, Dr. Ho and his team have made seminal contributions to understanding the biochemical and immunological properties of glypicans, including GPC1, GPC2, and GPC3, in cancer development and immunotherapy. His lab has advanced knowledge of glypicans in Wnt and YAP signaling, their structure-function relationships, and the discovery of previously unidentified associated proteins, such as FAT1 and AFP. They have also developed antibody- and T-cell-based immunotherapies for the treatment of liver cancer, mesothelioma, pediatric cancers, and other malignancies. Additionally, Dr. Ho's lab develops antibody engineering tools, such as mammalian cell surface display technology and shark and camel nanobody libraries, to advance drug discovery. Some of their research has direct clinical applications.
Developing glypicans as cancer therapeutic targets: structure and function
Antibody-based therapy has demonstrated promising efficacy in hematologic tumors, but it has encountered limitations in the context of most solid cancers. One of the primary challenges lies in the scarcity of cancer-specific antigens across many solid tumors. Our research focus centers on the biochemistry and cell biology of cell surface receptors, including cell surface glypicans, with the aim of developing them as novel targets for combating cancer.
Heparan and chondroitin sulfate proteoglycans (HSPGs and CSPGs) serve as critical modulators of signal transduction pathways during development and disease. HSPGs predominantly comprise glycosylphosphatidylinositol (GPI)-anchored glypicans and transmembrane syndecans. In the realm of human glypicans, six members (GPC1 to GPC6) can be categorized into two overarching subfamilies: GPC1/2/4/6 and GPC3/5. A significant portion of our work delves into the fundamental aspects of glypicans, particularly their connections to the Wnt, Yap, and other oncogenic signaling pathways. Our overarching objective is to investigate the potential of glypicans, such as GPC1, GPC2, and GPC3, as promising therapeutic targets in the realm of cancer.
GPC3, highly expressed in over 70% of hepatocellular carcinoma, a prevalent type of primary liver cancer, demonstrates negligible expression in normal adult tissues. In our research, we have identified two functional Wnt binding sites on GPC3, one situated within the protein core and the other within the heparan sulfate chains. Collaboratively with Byungkook Lee (NCI), we have developed a structural model of the GPC3/Wnt complex and experimentally delineated the hydrophobic groove in the N-lobe of the protein core of GPC3, thus furnishing evidence for GPC3's role as a co-receptor in the regulation of Wnt signaling. Moreover, we have discovered that a single-domain antibody (HN3) can effectively inhibit Wnt/β-catenin signaling by binding to the Wnt functional binding groove on GPC3. Additionally, our HS20 human antibody, specific for the heparan sulfate chains of GPC3, has been instrumental in defining the Wnt binding motif, which likely functions as a Wnt storage/transporter site on the heparan sulfate chains. In collaboration with Jian Liu (University of North Carolina), we have established that Wnt recognizes a heparan sulfate motif containing IdoA2S and GlcNS6S, with 3-O-sulfation in GlcNS6S3S enhancing Wnt binding. Our research has further unveiled the pivotal role of GPC3 in regulating Yap signaling for cancer cell proliferation and has identified an interaction between GPC3 and HGF in liver cancer. Most recently, we have identified FAT1, a potential cell surface receptor of Yap signaling in mammalian cells, as a novel GPC3-interacting protein in liver cancer cells. We have also identified alpha-fetoprotein (AFP) as a GPC3-associated protein. Both GPC3 and AFP are oncofetal proteins and important biomarkers in liver cancer.
In recent years, we have applied the knowledge gained from our research on GPC3 to explore the role of other glypicans in cancer. Notably, we discovered that GPC2 protein expression was evident in nearly half of neuroblastoma cases. Inactivating GPC2 through knockout led to the suppression of Wnt/β-catenin signaling and a reduction in the expression of N-Myc, a critical driver of neuroblastoma tumorigenesis. Building on this finding, we conducted a proof-of-concept study demonstrating that CAR T cells and immunotoxins targeting GPC2 could effectively hinder neuroblastoma growth in mouse models. Consequently, we established GPC2 as a novel therapeutic target for neuroblastoma.
GPC1, found to be overexpressed in various cancer types, including pancreatic cancer, exerts influence over multiple signaling pathways, including Wnt. In our investigation of GPC1's role in Wnt signaling in pancreatic cancer cells, we examined the expression of Wnt3a. The results revealed heightened levels of Wnt3a protein in nearly all pancreatic cancer cell lines compared to normal pancreatic duct cells. To further assess GPC1's impact on Wnt signaling, we overexpressed GPC1 in HEK293 SuperTopflash cells, which feature a luciferase reporter under the control of three LEF/TCF binding sites. Upon exposure to Wnt3a conditioned medium, we observed that GPC1 overexpression significantly induced Wnt activation to a similar extent as GPC3 overexpression. Utilizing our D4 nanobody against GPC1, we constructed immunotoxins and CAR-T cells. The immunotoxin effectively inhibited pancreatic tumor growth by facilitating the degradation of internalized GPC1, downregulating Wnt signaling, and inhibiting protein synthesis. Notably, GPC1 exhibited a substantially shorter intracellular half-life (15 minutes) compared to GPC2 (1 hour) and GPC3 (2 hours), which are other members of the glypican family. Treatment with immunotoxins reduced the expression levels of the short-lived GPC1 through lysosomal degradation following internalization. This downregulated GPC1 subsequently inhibited the downstream Wnt signaling cascade by reducing active β-catenin levels. Furthermore, GPC1 CAR-T cells significantly downregulated β-catenin expression in pancreatic cancer cells, suggesting that they may downregulate Wnt/β-catenin signaling in target pancreatic tumor cells. These findings underscore GPC1 as a promising therapeutic target in pancreatic cancer.
Mesothelin is highly expressed in various solid tumors, including mesothelioma and ovarian cancer. In our quest to understand its biological function, we collaborated with Byungkook Lee (NCI) to create a structural model and experimentally identify the functional binding domain for MUC16/CA125 (residues 296-359, designated as IAB) at the N-terminus of cell surface mesothelin. Working alongside Ira Pastan (NCI) and Manish Patankar (University of Wisconsin-Madison), we established that the MUC16-associated N-glycans are essential for binding to mesothelin. Our research elucidates the molecular mechanisms through which mesothelin regulates MUC16/CA125 in the context of cancer pathogenesis.
Engineering antibody therapeutics for treating cancer: immunotoxins and CAR T cells
To address the limitations of antibody-based immunotherapy in the context of solid tumors, we've harnessed various antibody technologies to create extensive libraries of human and xenogeneic antibodies. We've also employed multiple effector mechanisms to enhance the anti-tumor efficacy of our tumor antigen-specific monoclonal antibodies. Our research program is dedicated to the development and application of antibody-based immunotherapeutic strategies for treating solid tumors, including hepatocellular carcinoma (HCC), pancreatic cancer, neuroblastoma, and mesothelioma. The strategies developed within our program hold promise for potential application in the treatment of other solid tumors.
To validate GPC3 as a therapeutic target for cancer, we've generated antibodies, namely HN3 and YP7, that specifically bind to GPC3 on liver cancer cells. HN3, a human single-domain antibody obtained through phage display technology, targets a concealed Wnt binding site in the N-lobe of GPC3. YP7, on the other hand, is a monoclonal antibody isolated using mouse hybridoma technology, and it binds to an epitope near the cell surface at the C-lobe of GPC3. Leveraging these antibodies, we've developed immunotoxins and antibody-drug conjugates (ADCs) to target GPC3-expressing cancers. Our innovative immunotoxin operates through a dual mechanism of action, effectively deactivating cancer signaling pathways (Wnt and Yap) through the antibody domain and inhibiting protein synthesis via the toxin component. This dual-action approach holds the potential to enhance the efficacy of other antibody-toxin/drug conjugates for anti-tumor purposes. Nonetheless, the immunogenicity and short serum half-life of immunotoxins may pose limitations in clinical translation. To address these challenges, we've collaborated with Ira Pastan (NCI) to engineer immunotoxins based on HN3, incorporating various deimmunized Pseudomonas exotoxin (PE) domains and adding a single domain for albumin binding. Additionally, we've designed CAR T cells utilizing our GPC3 antibodies, and our humanized YP7 (hYP7) antibody-derived CAR T cells have exhibited potent and sustained anti-tumor activity in orthotopic liver cancer mouse models. The clinical trial using these hYP7 CAR T cells for the treatment of liver cancer patients is being led by our collaborator, Tim Greten (NCI).
Through RNA-seq analysis, we've demonstrated that exon 3 and exons 7-10 of GPC2 are significantly expressed in cancer but minimally in normal tissues. Consequently, we've identified a monoclonal antibody (CT3) that recognizes exons 3 and 10 and visualized the complex structure of CT3 and GPC2 through electron microscopy. This approach has been exemplified by the development of CT3-derived CAR T cells, which have shown efficacy in regressing neuroblastoma in mouse models. Genomic sequencing of T cells recovered from mice has revealed the integration sites of CARs, contributing to CAR T cell proliferation and persistence. These studies showcase how RNA-seq data can aid in identifying tumor-associated exons that are potential targets for CAR T cell therapies. Currently, CT3-derived CAR T cells are in development for a clinical trial at the NIH aimed at treating neuroblastoma patients through collaboration with the Pediatric Oncology Branch at the NCI.
Mesothelin, highly expressed in mesothelioma and other major solid tumors, has been the focus of our research. We previously isolated HN1, a human monoclonal antibody that disrupts the mesothelin-MUC16 interaction. Our recent efforts have centered on targeting the membrane-proximal region of mesothelin to enhance anti-tumor activities. As a result, we've isolated YP218, a unique rabbit monoclonal antibody targeting the C-terminal portion of mesothelin near the tumor cell surface. Through collaboration with Raffit Hassan (NCI), we've developed CAR T cells based on humanized YP218, demonstrating their remarkable efficacy against mesothelin-positive tumors in mouse models.
The B7-H3 transmembrane protein is an emerging target that features two distinct epitope motifs, IgC and IgV, within its ectodomain. We've generated dromedary camel nanobodies targeting B7-H3 and have shown that CAR-T cells, based on nanobodies recognizing the IgC domain (but not IgV), exhibit potent anti-tumor activity against large tumors in mice. These CAR-T cells are characterized by highly activated T cell signaling and substantial tumor infiltration. Our findings emphasize the critical role of the specific target antigen epitope in governing optimal CAR-T activity and offer a nanobody-based B7-H3 CAR-T product for use in solid tumor therapy.
In efforts to optimize CAR-T cell therapy for solid tumors, we've utilized the D4 nanobody targeting GPC1 and the HN3 nanobody targeting GPC3. Our findings indicate that a structurally rigid IgG4H and CD28TM domain brings the two nanobodies into close proximity, promoting CAR dimerization, enhancing T-cell signaling, and leading to tumor regression even in the presence of low antigen density in mice. Additionally, our single-cell-based proteomic and transcriptomic analysis of CAR T cells has identified specific genes associated with high CAR-T cell "polyfunctionality" in animal models. Future studies based on these findings will contribute to the development of more potent and safer CAR-T cell therapy for cancer treatment.
Developing antibody engineering technology: mammalian cell surface display and single domain antibodies
We have developed various antibody engineering technologies to produce therapeutic antibodies. These technologies encompass the development of mammalian cell surface display, the construction of shark and camel single-domain antibody phage libraries, and humanization of rabbit antibodies. These antibody engineering tools, including single-domain antibody libraries, can be utilized for the discovery of therapeutic drugs and diagnostic agents targeting human diseases such as cancer, infectious diseases, and neurological disorders.
Single-domain antibodies, commonly referred to as nanobodies, are known for their ability to bind to restricted epitopes that may be inaccessible to conventional antibodies. To create a large and diverse single-domain phage library, we introduced a method called PCR-Extension Assembly and Self-Ligation (EASeL) to construct a VNAR single-domain antibody library from nurse sharks (Ginglymostoma cirratum) in collaboration with Martin Flajnik (University of Maryland). Subsequently, we conducted next-generation sequencing analysis of 1.2 million shark VNAR single domains, demonstrating the high diversity of our shark single-domain library. Moreover, we applied the EASeL method to construct VHH single-domain phage libraries from dromedary camels (Camelus dromedaries). Single-domain antibodies isolated from these phage libraries exhibit high affinity (KD < 1 nM) for their respective tumor or viral antigens. These single-domain antibodies have been employed in the engineering of CAR-T cells, immunotoxins, and other therapeutic platforms for cancer treatment.
Antibody engineering traditionally involves displaying antibody fragments on the surface of microorganisms (e.g., phage, bacteria, and yeast). We have introduced a novel antibody engineering method known as 'mammalian cell display,' which is adapted from yeast display. With this approach, antibody fragments are expressed on mammalian cells, and high-affinity antigen binders are isolated from a combinatorial library using flow cytometry.
In the pursuit of developing rabbit antibodies for clinical applications, we conducted a comprehensive analysis of the complex structures of rabbit antibodies in conjunction with their antigens obtained from the Protein Data Bank. This analysis allowed us to identify antigen-contacting residues on the rabbit Fv within a 6 Angstrom distance from the antigen. Building on this structural insight, we devised a humanization strategy by grafting the structure-based CDRs into a human germline framework sequence. Immunotoxins composed of the humanized rabbit Fvs (e.g., hYP218) fused with clinically used toxins exhibited greater cytotoxicity against tumor cells compared to immunotoxins derived from their original rabbit Fvs. CAR T cells based on the hYP218 antibody demonstrated effective inhibition of tumor growth in mice. This method can serve as a general approach to humanize rabbit antibodies.
Drug resistance is a critical aspect of tumor biology that demands a complex cellular environment for study. In collaboration with Shuichi Takayama (University of Michigan) and V. Courtney Broaddus (UCSF), we've established ex vivo tumor spheroid models utilizing both cell lines and primary patient cells. These models enable the study of the molecular mechanisms of drug resistance within a physiologically relevant cellular framework. Additionally, we've employed microarrays to profile gene expression in both spheroids and monolayers, leading to the identification of new targets specific to the 3D biological structure of cancer.
Discovering antibody drugs for treating COVID-19: nanobodies broadly neutralizing SARS-CoV-2 variants
Severe acute respiratory syndrome coronavirus 2 (SARS-CoV-2) is the etiologic agent of COVID-19, which enters human cells by binding its envelope-anchored type I fusion protein (spike) to angiotensin-converting enzyme 2 (ACE2). Numerous neutralizing antibodies targeting the spike, particularly its ACE2 receptor binding domain (RBD), have been developed to treat COVID-19 using common strategies such as single B cell cloning, animal immunization, and phage display. However, SARS-CoV-2 variants, such as D614G, B.1.1.7, B.1.351, P.1, and B.1.617.2, have exhibited increased resistance to neutralization by monoclonal antibodies. Highly effective and broadly neutralizing antibody therapy is in demand for COVID-19 patients worldwide.
Due to their small size, nanobodies can recognize protein cavities that are not accessible to conventional antibodies. Dromedary camels have been found as potential natural reservoirs of MERS-CoV. We speculated that dromedary camels would be an ideal source of neutralizing nanobodies against coronaviruses. Using our dromedary camel (Camelus dromedarius) VHH phage libraries, we successfully discovered potent neutralizing nanobodies against SARS-CoV-2 variants. In collaboration with Mario Borgnia (NIEHS) and Raul Cachau (NCI), we solved the cryo-EM complex structures that reveal one VHH nanobody (8A2) binds the S1 subunit of the viral spike protein, and the other (7A3) targets a deeply buried region that uniquely extends to the S2 subunit beyond the S1 subunit. In collaboration with Hang Xie (FDA), we found that these nanobodies could protect mice from the lethal challenge of variants B.1.351 or B.1.617.2, suggesting the therapeutic potential of these nanobodies against COVID-19. We have also isolated shark VNARs that bind to the S2 subunit by phage panning from a naïve nurse shark VNAR phage display library constructed in our laboratory. Among those binders, S2A9 showed the best neutralization activity against the original pseudotyped SARS-CoV-2 virus. Several binders, including S2A9, showed cross-reactivity against S2 subunits from other β coronaviruses. Furthermore, S2A9 showed neutralization activity against all variants of concern (VOCs) from alpha to omicron (including BA1, BA2, BA4, and BA5) in both pseudovirus and live virus neutralization assays. Our findings suggest that S2A9 could be a promising lead molecule for the development of broadly neutralizing antibodies against SARS-CoV-2 and emerging variants. Our dromedary camel VHH libraries and nurse shark VNAR phage library offer a novel platform that can be used to rapidly isolate single-domain antibodies against emerging viral pathogens.
Teaching Interests
BIOC301/302 - Biochemistry I/II
Biography
Dr. Ho received a B.S. in Biology from East China Normal University and an M.A. in Cellular and Molecular Biology from San Francisco State University. He completed his Ph.D. in Immunology at the University of Illinois at Urbana-Champaign with an NIH NRSA F31 predoctoral fellowship. Dr. Ho then joined the National Cancer Institute (NCI) for a postdoctoral fellowship under Ira Pastan. He was appointed a tenure-track Investigator in 2008 and promoted to Senior Investigator with tenure in 2015. He currently serves as Deputy Chief of the Laboratory of Molecular Biology and Director of the Antibody Engineering Program at the NCI.
Dr. Ho is the founding Editor-in-Chief of Antibody Therapeutics (Oxford University Press) and serves on the boards of directors for the Antibody Society and the Foundation for Advanced Education in the Sciences. He received the APAO Scientific Achievement Award, the Dr. Francisco S. Sy Award for Mentorship Excellence at HHS, the NIH DDIR Innovation Award, and the NCI Director's Innovation Award. Dr. Ho was elected a Fellow of the American Institute for Medical and Biological Engineering (AIMBE) (2023) and named the Asian American Engineer of the Year (2024).
Selected Publications
- Li N, Quan A, Li D, Pan J, Ren H, Hoeltzel G, de Val N, Ashworth D, Ni W, Zhou J, Mackay S, Hewitt SM, Cachau R, Ho M. The IgG4 hinge with CD28 transmembrane domain improves V(H)H-based CAR T cells targeting a membrane-distal epitope of GPC1 in pancreatic cancer. Nat Commun. 2023;14(1):1986.
- Li N, Torres MB, Spetz MR, Wang R, Peng L, Tian M, Dower CM, Nguyen R, Sun M, Tai CH, de Val N, Cachau R, Wu X, Hewitt SM, Kaplan RN, Khan J, St Croix B, Thiele CJ, Ho M. CAR T cells targeting tumor-associated exons of glypican 2 regress neuroblastoma in mice. Cell Rep Med. 2021;2(6):100297.
- Li D, Li N, Zhang YF, Fu H, Feng M, Schneider D, Su L, Wu X, Zhou J, Mackay S, Kramer J, Duan Z, Yang H, Kolluri A, Hummer AM, Torres MB, Zhu H, Hall MD, Luo X, Chen J, Wang Q, Abate-Daga D, Dropulic B, Hewitt SM, Orentas RJ, Greten TF, Ho M. Persistent Polyfunctional Chimeric Antigen Receptor T Cells That Target Glypican 3 Eliminate Orthotopic Hepatocellular Carcinomas in Mice. Gastroenterology. 2020;158(8):2250-2265.e20.
- Li N, Fu H, Hewitt SM, Dimitrov DS, Ho M. Therapeutically targeting glypican-2 via single-domain antibody-based chimeric antigen receptors and immunotoxins in neuroblastoma. Proc Natl Acad Sci U S A. 2017;114(32):E6623-E6631.
- Feng M, Gao W, Wang R, Chen W, Man YG, Figg WD, Wang XW, Dimitrov DS, Ho M. Therapeutically targeting glypican-3 via a conformation-specific single-domain antibody in hepatocellular carcinoma. Proc Natl Acad Sci U S A. 2013;110(12):E1083-91.
Related Scientific Focus Areas
Biomedical Engineering and Biophysics
View additional Principal Investigators in Biomedical Engineering and Biophysics
Molecular Biology and Biochemistry
View additional Principal Investigators in Molecular Biology and Biochemistry
This page was last updated on Tuesday, May 27, 2025