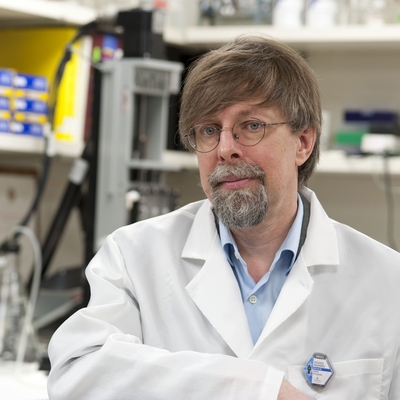
Research Topics
PROJECTS
1. Control of transcription elongation and transcription fidelity
In the cell, information encoded in the DNA is faithfully transferred to protein by mRNA intermediate generated by RNA polymerases. Due to the transient nature of RNA, the biological importance of transcription fidelity has been notoriously difficult to investigate. The error-prone transcription has been proposed to contribute to cancer, aging and adaptive mutagenesis. Genetic selections and screens performed with libraries of randomly mutagenized subunits of RNA polymerase II (Pol II) from Saccharomyces cerevisiae (S. cerevisiae) and Escherichia coli (E. coli) RNA polymerase revealed mutations that increase the frequency of NTP mis-incorporation and insertions/deletions (slippage) in mRNA. Biochemical analysis of these mutants confirmed the low fidelity phenotype of the mutant polymerases. Insertion and deletion mutations generated by transcription slippage also have impact on biology because they result in translational frame-shifts and lead to synthesis of truncated proteins potentially toxic to the cell. In addition, transcription errors introduce a major disturbance to the active site of RNA polymerase leading to a prolonged pausing/arrest of the enzyme on DNA to interfere with gene expression and DNA replication. Increased misincorporation was not originally expected to have a profound biological effect. However, the biology-driven approach revealed mutations that render yeast dependent on error-correction protein factor TFIIS, make the cells sensitive to NTP depletion by 6-azauracil and increase misincorporation by Pol II in vitro. Taken together, these observations justified using the error-prone Pol II and E. coli RNA polymerase as a tool to develop screening approaches for mutants with increased errors. My laboratory performs biochemical characterization of the polymerase mutants that are error-prone in vivo to confirm increased misincorporation and slippage. We identify protein domains in RNA polymerase subunits responsible for control of transcription fidelity. In collaboration with Jeff Strathern, Donald Court, and Ding Jin’s groups in the Gene Regulation and Chromosome Biology Laboratory, we develop highly sensitive fidelity Cre/lox reporter systems capable of detecting infrequent transcription errors in E. coli and yeast. These reporters allow us to test the old-standing question of whether transcription errors fluctuate in a broad range depending on growth conditions and upon variable cellular stresses.
2. Direct assessment of transcription fidelity by high-resolution RNA sequencing (RNA-seq and NET-seq)
Cancerous and aging cells have long been thought to be impacted by transcription errors that cause genetic and epigenetic changes. Until now a lack of methodology for directly assessing such errors hindered evaluation of their impact to the cells. We have developed a high-resolution Illumina RNA-seq method that can assess non-coded base substitutions in mRNA at 10.000-100.000 per base frequencies in vitro and in vivo. Statistically reliable detection of changes in transcription fidelity assures that the RNA-seq can analyze transcription errors in a large number of genomic sites. A combination of the RNA-seq and biochemical analyses of the positions for the errors revealed two sequence-specific mechanisms that increase transcription fidelity by Escherichia coli RNA polymerase: (i) enhanced suppression of nucleotide misincorporation that improves selectivity for the cognate substrate, and (ii) increased backtracking of the RNA polymerase that decreases a chance of error propagation to the full-length transcript after misincorporation and provides an opportunity to proofread the error. The high-resolution RNA-seq is adoptable to a genome-wide assessment of transcription fidelity and post-transcriptional RNA editing far beyond E. coli cells. To complement the RNA-seq, we employ an improved method of the nascent elongating transcript sequencing (NET-seq) to study transcription errors in E. coli and yeast cells. On that avenue, we analyze biological impacts of transcription errors to genome stability in the single cell organisms (E. coli and yeast) followed by implementation of these findings and techniques to mammalian cells.
3. Role of translesion transcription in transcription-coupled DNA repair and in cellular resistance to DNA damage
The faithful and efficient transcription of genomic DNA into mRNA is crucial for cell survival under DNA damage caused by UV irradiation, oxidative stress, or chemical DNA modifications. To maintain genomic integrity, cells have evolved separate cellular strategies involving multiple DNA damage repair and DNA damage tolerance mechanisms. Non-bulky DNA lesions are preferentially repaired by the base excision repair (BER) pathway. The damages that cause large DNA distortion, such as UV light-induced cyclobutane pyrimidine dimers/cisplatin adducts and oxidative cyclopurines are primarily subjected to the nucleotide excision repair (NER) pathway. Despite ongoing repair, some lesions escape detection, presenting the cell with a challenge for continued DNA and RNA synthesis. During replication, the deleterious effect of DNA lesions can be alleviated by translesion DNA synthesis (TLS). During TLS, the high-fidelity replicative DNA polymerases are switched transiently to specialized translesion DNA polymerases that can accommodate bulky lesions within a more spacious active site, thus enabling their bypass. Our work on transcription-coupled DNA repair (TCR) revealed that yeast Pol II has an intrinsic capability to bypass UV-induced pyrimidine dimers as an alternative pathway to its preferential repair by TC-NER. We defined this process as RNA polymerase translesion synthesis (RTLS) to distinguish it from the regular TLS by DNA polymerases. We further demonstrated that the efficiency of the pyrimidine dimers bypass by Pol II correlates with increased UV cell resistance. Most importantly, our most recent results revealed that mammalian Pol II employs the similar mechanism to negotiate with the pyrimidine dimers in vitro. To address the functional correlation between RTLS and TC-NER, we investigate the mechanism for strand specific repair in various genetic backgrounds in yeast as a model system. We expand our effort to transcription across the other types of DNA lesions including cyclopurine lesions (cyclo-dA and cyclo-dG) and 8-oxoguanine. We showed that yeast and mammalian Pol II are capable of transcription through cyclo-dA in vitro by using a mechanism, which is strikingly similar to transcription across the pyrimidine dimers.
As a separate effort, we continue elucidation of the role of Rpb4/7 and Rpb9 subunits of Pol II in initiation of TCR and will search for repair proteins specific to Rpb4/7- and Rpb9-dependent TCR pathways. In the long term, we aim to evaluate an impact of faithful and error-prone transcription to genome integrity during normal growth and endogenous/exogenous stresses caused by DNA damage, tumorigenesis and aging.
4. Mechanisms of transcription through nucleosomes by RNA polymerase II
This theme addresses the intriguing question of how eukaryotic RNA polymerases transcribe through chromatin. The project involves development of the minimal in vitro system for transcription through nucleosome by Pol II and Pol III from S. cerevisiae. RNA polymerase from E. coli, which behaves very similarly to the eukaryotic enzyme in nucleosomal transcription, is used in this project as an easy-handled prototype of Pol II. Is it related at all to cancer in humans? Yes, it is. A central characteristic of cancer is deregulation of transcription control leading to activation of expression of growth-promoting genes, as well as silencing of genes with the tumor suppressor functions. Importantly, mutations found in tumor cells cannot alone explain the complexity of the change in pattern of gene expression. Epigenetic changes in the transcribed regions, such as DNA methylation, covalent modifications of the histones, and ATP-dependent chromatin remodeling have been recently identified as key universal components of the transformation process. Therefore, epigenetic changes are considered promising targets for new anti-cancer drugs. However, development of the novel therapies is significantly hampered by the complexity of the interplay between the different pathways of epigenetic modifications. Rapid progress in this field depends on the understanding of the molecular mechanism of action of each of the epigenetic modifications, and establishment of hierarchy of the events ultimately leading to alterations of gene expression. To predict the effect of a certain epigenetic modification on the level of expression of a given gene, it is necessary to understand how the changes in chromatin, caused by remodeling and modification, affect transcription. Pol II transcribes templates packaged into the nucleosomes. Several lines of experimental evidence suggest that chromatin remodeling and modification occurs during promoter activation, and is associated with transcription elongation. It is not known if any chromatin remodeling and modification events are generally required for efficient elongation, and which play the gene-specific regulatory roles. To understand the molecular mechanism of transcription on chromatin templates by Pol II in vivo, we determine how the polymerase transcribes through a single nucleosome and short nucleosomal arrays in vitro.
Biography
Dr. Kashlev received his Ph.D. in molecular biology from Institute of Molecular Genetics, Moscow in 1990. He was a postdoctoral fellow in the Department of Microbiology at Columbia University from 1991 to 1992 and a research associate at the Public Health Research Institute from 1993 to 1996. In 1996, Dr. Kashlev joined the ABL-Basic Research Program and established the Molecular Mechanisms of Transcription Section. In 1999, he joined the Center for Cancer Research and was tenured in 2007.
Selected Publications
- Walmacq C, Wang L, Chong J, Scibelli K, Lubkowska L, Gnatt A, Brooks PJ, Wang D, Kashlev M. Mechanism of RNA polymerase II bypass of oxidative cyclopurine DNA lesions. Proc Natl Acad Sci U S A. 2015;112(5):E410-9.
- Imashimizu M, Takahashi H, Oshima T, McIntosh C, Bubunenko M, Court DL, Kashlev M. Visualizing translocation dynamics and nascent transcript errors in paused RNA polymerases in vivo. Genome Biol. 2015;16:98.
- Kakar S, Fang X, Lubkowska L, Zhou YN, Shaw GX, Wang YX, Jin DJ, Kashlev M, Ji X. Allosteric Activation of Bacterial Swi2/Snf2 (Switch/Sucrose Non-fermentable) Protein RapA by RNA Polymerase: BIOCHEMICAL AND STRUCTURAL STUDIES. J Biol Chem. 2015;290(39):23656-69.
- Irvin JD, Kireeva ML, Gotte DR, Shafer BK, Huang I, Kashlev M, Strathern JN. A genetic assay for transcription errors reveals multilayer control of RNA polymerase II fidelity. PLoS Genet. 2014;10(9):e1004532.
- Imashimizu M, Kireeva ML, Lubkowska L, Gotte D, Parks AR, Strathern JN, Kashlev M. Intrinsic translocation barrier as an initial step in pausing by RNA polymerase II. J Mol Biol. 2013;425(4):697-712.
Related Scientific Focus Areas
Molecular Biology and Biochemistry
View additional Principal Investigators in Molecular Biology and Biochemistry
This page was last updated on Thursday, September 19, 2024