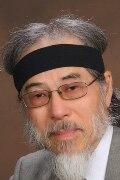
Kiyoshi Mizuuchi, Ph.D.
NIH Distinguished Investigator
Genetic Mechanisms Section, Laboratory of Molecular Biology
NIDDK
Research Topics
The objective of our research is to gain insights into the mechanics of cellular processes that impact the genomic structure and the heritance of the genetic material by progenies of proliferating cells. Previously, we have studied mechanisms of DNA rearrangement reactions that impact the stability of the linear organization of the genome. More recently, we have focused our attention to understand the mechanisms of bacterial cellular processes that ensure replicated copies of chromosomes are partitioned into two dividing daughter cells.
Chromosome segregation and cell division in bacteria
After DNA replication, two copies of the bacterial chromosome and low copy number plasmids must be segregated into two daughter cells to ensure inheritance. Therefore, systems have evolved to actively partition the replicated copies of the genome to two halves of the cell before cell division takes place. One commonly found class of such systems involve three components: a specific DNA sequence on the segregating chromosome that functions as the bacterial equivalent of a “centromere”, a protein factor that binds to the “centromere”, and a second protein factor, an ATPase with ATP-dependent nonspecific DNA-binding activity. E. coli P1-plasmid and F-plasmid are equipped with such systems. We have reconstituted cell-free reaction systems to study these plasmid DNA partition reactions in a flow cell under a microscope. Based on the experimental results we obtained by combining biophysical and biochemical approaches, we have uncovered a previously unappreciated biological cargo transport and positioning mechanism that involves a variation of the reaction-diffusion principle, which we call diffusion-ratchet mechanism.
After segregation of replicated chromosome and plasmid copies to two halves of the cell, cell division normally must take place at the mid-cell to produce similarly sized daughter cells. We study the mechanism of mid-cell localization of the cell division septum in E. coli, which is controlled by a set of Min-proteins, with biophysical techniques making use of a reconstituted cell-free reaction system observed by fluorescent microscopy. The position of cell division septum formation is determined by the location where a bacterial tubulin-homologue, FtsZ polymerizes on the inner membrane to lay the foundation for the cell division machinery. FtsZ polymerization does not take place everywhere on the membrane, because it is controlled by multiple systems, one of which is the Min system. Here, FtsZ polymerization is inhibited by membrane-localized MinC protein. MinC is brought to membrane by binding to MinD, an ATP-dependent membrane binding protein. Together with MinE, a stimulator of MinD ATPase, Min proteins self-organizes cell pole to cell pole standing wave-type oscillation on the membrane inside a cell, generating time-averaged concentration minimum of MinD and MinC at mid-cell, confining cell division there by inhibiting division elswhere. We have successfully recapitulated the standing-wave-type oscillatory dynamic pattern self-organization by MinD/E proteins in a cell-free reaction. Based on our findings, we now have a detailed mechanistic model of the system, which will be subjected to further experimental tests and refinements.
Genome rearrangement by transpositional recombination
The genomes of all organisms are under the threat of assault by transposable elements. We have studied the mechanisms of DNA transposition of the bacteriophage Mu as a model system for a wide family of DNA rearrangement reactions from bacteria to humans. These reactions are involved in many processes that impact our health, from the spread of drug resistance among pathogenic bacteria to replication of retroviruses such as HIV. The immunoglobulin gene rearrangement reaction in vertebrates also takes place by a closely related mechanism. By using biochemical, molecular biological, and biophysical approaches, including single-molecule techniques, we have advanced our understanding of the reaction steps and the chemical mechanisms involved in these reactions. Certain bacterial transposons including phage Mu selectively transpose to target DNA sites away from where a pre-existing copy of the same transposon is located. This self-avoidance process, called transposition target immunity, in the case of the Mu system, involves along with the tansposase protein, MuA, which plays central roles in the transpositional recombination steps, a second protein MuB. MuB is an ATP-dependent non-specific DNA binding protein, whose ATPase activity is stimulated by MuA. While we were making progress on our understanding of this target site selection system, Barbara Funnell at University of Toronto alerted us on the biochemical parallel of this two-protein system and the P1 plasmid partition system she studies. This insight of hers led to our collaboration and our additional current projects described above.
Applying our Research
Advances of molecular understanding of specific reaction systems we study that are essential for efficient bacterial growth could provide a basis for the future development of novel antibacterial agents to combat disease-causing bacteria.
In a broader sense, progress in these projects is expected to advance our general mechanistic understanding of cellular processes such as biomolecular cargo transport and positioning systems and broad range of meso-scale biological positional control mechanisms that operate at much larger length scale than individual molecular components such as protein molecules that constitute the system.
Biography
- D.S. (Ph.D.), Osaka University, 1972
- M.S., Osaka University, 1969
- B.S., Osaka University, 1967
Selected Publications
- Vecchiarelli AG, Neuman KC, Mizuuchi K. A propagating ATPase gradient drives transport of surface-confined cellular cargo. Proc Natl Acad Sci U S A. 2014;111(13):4880-5.
- Vecchiarelli AG, Li M, Mizuuchi M, Hwang LC, Seol Y, Neuman KC, Mizuuchi K. Membrane-bound MinDE complex acts as a toggle switch that drives Min oscillation coupled to cytoplasmic depletion of MinD. Proc Natl Acad Sci U S A. 2016;113(11):E1479-88.
- Taylor JA, Seol Y, Budhathoki J, Neuman KC, Mizuuchi K. CTP and parS coordinate ParB partition complex dynamics and ParA-ATPase activation for ParABS-mediated DNA partitioning. Elife. 2021;10.
- Hwang LC, Vecchiarelli AG, Han YW, Mizuuchi M, Harada Y, Funnell BE, Mizuuchi K. ParA-mediated plasmid partition driven by protein pattern self-organization. EMBO J. 2013;32(9):1238-49.
- Han YW, Mizuuchi K. Phage Mu transposition immunity: protein pattern formation along DNA by a diffusion-ratchet mechanism. Mol Cell. 2010;39(1):48-58.
Related Scientific Focus Areas
Molecular Biology and Biochemistry
View additional Principal Investigators in Molecular Biology and Biochemistry
Microbiology and Infectious Diseases
View additional Principal Investigators in Microbiology and Infectious Diseases
This page was last updated on Wednesday, January 10, 2024