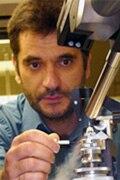
Frederick P. Dyda, Ph.D.
Senior Investigator
Structural Biochemistry Section, Laboratory of Molecular Biology
NIDDK
Research Topics
p { margin-bottom: 0.1in; direction: ltr; line-height: 120%; text-align: left; widows: 0; orphans: 0 } a:link { so-language: zxx }
The goal of our research is to understand how some segments of DNA, called mobile genetic elements (MGE), move within cells and between cells. In general, the reorganization of genomes by MGEs has had a huge impact on the evolution of many organisms. They are one of the drivers of the emergence of antibiotic resistance. Importantly, they can be engineered and used as tools for a variety of genomic applications, including gene therapy.
Current Research
p { margin-bottom: 0.1in; direction: ltr; line-height: 120%; text-align: left; widows: 0; orphans: 0 } a:link { so-language: zxx }
The current focus of the lab is to understand the molecular details of how certain MGEs work and armed with this knowledge to redesign them so they can be part of our gene therapy toolkit. To mobilize DNA, most MGEs encode an enzyme called the transposase that orchestrates a series of molecular events such as DNA recognition, its cleavage, and integration, all mediated by a large nucleoprotein complex called the transpososome. We use a variety of sophisticated experimental and computational tools to understand these processes. In particular, to understand how they work, we use three dimensional structural information at the near-atomic resolution level of these complexes obtained either by single particle cryo-electron microscopy or X-ray diffraction, and a large array of computational tools for both modeling and structure prediction. To visualize the intricacies of their actions, we produce structural “snapshots” of these highly dynamic macromolecular assemblies as they proceed through the path that ends with the MGE integrated at a new genomic location. With these snapshots in hand, we use a variety of biochemical, biophysical, and computational approaches to bridge the structures and biological function and to generate and test ideas of their redesigns.
For more information, see my group on the Structural Biology Section home page.
Applying our Research
p { margin-bottom: 0.1in; direction: ltr; line-height: 120%; text-align: left; widows: 0; orphans: 0 } a:link { so-language: zxx }
One of the most exciting thing about MGEs is their potential application in gene therapy. Some MGEs can deliver large DNA segments and integrate these in the genomes of a variety of different cell types. A detailed mechanistic understanding of how they work could open up a variety of different avenues to modify and optimize MGEs so that clinicians will have them available in the future to treat patients using genetic tools.
Biography
- Ph.D., University of Pittsburgh, 1992
- B.S., Eötvös Lóránd University, 1986
Selected Publications
- Hickman AB, Ewis HE, Li X, Knapp JA, Laver T, Doss AL, Tolun G, Steven AC, Grishaev A, Bax A, Atkinson PW, Craig NL, Dyda F. Structural basis of hAT transposon end recognition by Hermes, an octameric DNA transposase from Musca domestica. Cell. 2014;158(2):353-367.
- Hickman AB, Kailasan S, Genzor P, Haase AD, Dyda F. Casposase structure and the mechanistic link between DNA transposition and spacer acquisition by CRISPR-Cas. Elife. 2020;9.
- Chen Q, Luo W, Veach RA, Hickman AB, Wilson MH, Dyda F. Structural basis of seamless excision and specific targeting by piggyBac transposase. Nat Commun. 2020;11(1):3446.
- Chappie JS, Acharya S, Leonard M, Schmid SL, Dyda F. G domain dimerization controls dynamin's assembly-stimulated GTPase activity. Nature. 2010;465(7297):435-40.
- Chappie JS, Mears JA, Fang S, Leonard M, Schmid SL, Milligan RA, Hinshaw JE, Dyda F. A pseudoatomic model of the dynamin polymer identifies a hydrolysis-dependent powerstroke. Cell. 2011;147(1):209-22.
Related Scientific Focus Areas
Molecular Biology and Biochemistry
View additional Principal Investigators in Molecular Biology and Biochemistry
This page was last updated on Wednesday, January 10, 2024