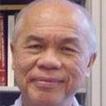
Research Topics
Key metabolic processes and cell signaling in biological systems are mediated by multiple well-regulated enzymatic activities. Dr. Chock’s laboratory employed biophysical and chemical methods to elucidate the kinetics and mechanisms of enzyme action and regulation, particularly those mediated by reversible covalent modification of proteins.
Based on detailed studies of the reversible adenylyltion of E. coli glutamine synthetase (GS), Dr. Chock, in collaboration with Dr. E. R. Stadtman, formulated a cyclic cascade model, to reveal the basic principles that underlie this regulatory mechanism and its regulatory advantages in cellular regulation and signal transduction, which include an enormous potential for signal and rate amplification, regulatory sensitivity, and flexibility.
In bacteria, GS links the assimilation of ammonia with diverse biosynthesis and leads to the synthesis of proteins, nucleic acids, complex polysaccharides, and coenzymes. Thus, it is rigorously regulated, including inactivation of the Mg(II)-bound GS mediated via adenylylation of Y-397 in each dodecameric subunit. Dr. Chock and his colleagues, particularly Dr. Sue Goo Rhee, elucidated the mechanism for both the catalytic action of E. coli GS and its regulation via a closed bicyclic cascade-catalyzed reversible adenylylation. The catalytic cycle monitored by both steady state and transient kinetic, EPR and fluorescent methods, reveal the formation of a GS-bound reactive intermediate, γ-glutamyl phosphate, which reacts with GS-bound ammonia to form its reaction products, glutamine. ADP and inorganic phosphate. Tyrosine adenylylation converts GS from a Mg(II)- to a Mn(II)-supported enzyme. Since Mn(II) concentration is relatively low under physiological conditions, adenylylation leads to GS inactivation. The reversible adenylation of GS is catalyzed by adenylyltransferase (AT), consisting of two catalytic sites, ATa and ATd, that catalyzes the adenylylation and deadenylylation of GS, respectively. Both activities of AT are regulated by another nucleotidylation cycle consisting of a bifunctional enzyme, uridylyltransferase (UT). The two catalytic sites of UT are Utu and Utd, that catalyzes the uridydylation and deuridydylation of a regulatory protein, PII, respectively. Uridylylated PII is required for ATd activity while unmodified PII stimulates the activity of ATa. These four interconvertible enzymes are regulated by multiple metabolites, particularly the enzymes that lead to GS inactivation are activated by glutamine and inhibited by α-ketoglutarate, a precursor of l-glutamine, while the activities that lead to the activation of GS are activated by α-ketoglutarate and inhibited by glutamine. Thus, the state of GS adenylylation varies rapidly in response to the availability of ammonia.
To address the activation mechanism and the regulatory advantages of having four Ca(II) binding sites on the calmodulin (CM), Dr. Chock and his colleagues, particularly Dr. Charles Huang, performed detailed kinetic analysis for Ca(II)CM mediated activation of cyclic nucleotide phosphodiesterase. They revealed that binding of four Ca(II) to CM is required to form an activated enzyme complex. Thus, the Ca(II)CM mediated activation of is highly cooperative with respect to Ca(II) concentration, and function as an effective on/off switch for enzyme activation. Their data revealed that CaCM elevates the affinity between the enzyme and the fully liganded CM by about 104 to 105 folds, a magnitude that can easily be achieved through a 10- to 20-fold increase in Ca(II) binding affinity for the enzyme-CM complex in each of the four binding steps With this affinity, the enzyme can be fully activated at free CM concentration of 0.1 to 10 nM during Ca surge. The four Ca binding-step also provides a relatively fast deactivation reaction.
To investigate the physiological roles of extreme form of negative cooperative enzymes, Dr. Chock and colleagues, particularly Dr. Charles Huang, investigated the catalytic action of E. coli alkaline phosphatase, a Zn(II) metalloenzyme consisting of two identical subunits. This dimeric enzyme exhibits half-of-the sites reactivity. To confer a catalytic role for the idle subunit of the dimer, an attractive Flip-Flop mechanism in which the two subunits serve alternately as the catalytic site was proposed by Lazdunski et al. However, steady-state and transient kinetic analysis revealed that the flip-flop mechanism is not supported by the expected Michaelis-Menten kinetic response, and binding of a second substrate molecule to facilitate product release in the flip-flop model is not detected. Nevertheless, we found that the strong negative cooperativity of alkaline phosphatase can be desensitized by a high level of Mg(II) concentration, and provides a regulatory role for obtaining a rapid surge in enzymatic activity.
To elucidate the mechanism by which Cu(II), Zn(II)-superoxide dismutase, an antioxidant enzyme, could exert a pro-oxidant activity, Dr. Chock and his colleagues, particularly, Dr. Moon Bin Yim, revealed that Cu(II), Zn(II)- superoxide dismutase possesses a free-radical generating activity detected with EPR-spin trap methods. This free radical generating activity is elevated in its familial amyotrophic lateral sclerosis-(FALS) associated A4V, and G93A mutants, due to the decrease of their Km values for hydrogen peroxide. In accord to this notion, free radical mediated mRNA oxidation was found to occur early in motor neuron deterioration in ALS.
In view of the fundamental importance of a proposed substrate channeling mechanism for direct transfer of metabolites from the producing enzyme to the utilizing enzyme in the glycolytic pathway, based on the kinetic interpretation that NADH is transferred directly between glycerol-3-phosphate dehydrogenase (GPDH) and lactate dehydrogenase (LDH), Dr. Chock and his colleagues, particularly Dr. Xiaomao Wu, developed a kinetic method based on theoretical analysis and kinetic patterns of the displacement reactions to differentiate a dissociative free diffusion mechanism from a substrate channeling mechanism. Their analysis revealed that in glycolysis, the transfer of NADH between GPDH and LDH proceeds via a dissociative and not a substrate channeling mechanism.
Growing evidence indicates that RNA oxidation is correlated with age-related neurodegenerative diseases. Dr. Chock and colleagues, particularly Dr. Mikiei Tanaka, investigated the mechanisms of RNA oxidation and their physiological consequences. They showed that moderately oxidized mRNA exhibits a significant reduction in its translation fidelity. In addition, the products of RNA oxidation can cross link with cytochrome c, and facilitate its release from mitochondria. Thus, RNA oxidation would work not only to impair normal RNA functions, but also invoke a protective signal to induce apoptosis in response to oxidative stress.
The involvement of bacteria in carcinogenesis remains controversial, in part, because there is no clear agreement on the molecular mechanism by which they can promote cancer development. Recently Dr. Chock and his colleagues, particularly, Dr. Hammou Oubrahim, investigated how cell signaling mechanisms mediated by bacterial toxins, e.g., Pasteurella Multocida toxin (PMT), might promote cancer. PMT is known for its potent mitogenic properties and its ability to induce anchorage-independent growth of certain cell types, a property with the potential to act as a tumor promoter, especially in the case of chronic infections. They have shown that PMT hijacks cell signaling pathways, in part via deamidation of a conserved glutamine residue in the α subunit of heterotrimeric G proteins and constitutively activates mTOR signaling via a Gαq/11/PLCβ/PKC mediated pathway. Furthermore, they showed that PMT upregulates biosynthesis of connective tissue growth factor (CTGF), and generates autocrine and/or paracrine signaling that leads to the activation of mTOR and MAPK.
Biography
P. Boon Chock received his Ph.D. in Chemistry from the University of Chicago in 1967. After postdoctoral training with Manfred Eigen at the Max-Planck-Institut fȕr biophysikaliche Chemie in Göttingen, Germany, he joined the Laboratory of Biochemistry, NHLBI, NIH in 1971 as a visiting scientist, He was converted to a research scientist in 1973, and promoted to the Chief of the Metabolic Regulation Section in 1981. Dr. Chock served as the Chief of the Laboratory of Biochemistry from 1994 to 2008, and as the Director of the Biochemistry and Biophysics Center from 2002 to 2008. He received the NIH Director’s Award in 1993. Dr. Chock has authored or coauthored 200 publications, including peer-reviewed manuscripts, review articles and book chapters. Dr. Chock has served in the editorial boards of the Journal of Biological Chemistry for more than 22 years, and of the Archives of Biochemistry and Biophysics, and BioFactors. He was the co-editor of Current Topics in Cellular Regulation Series from 1989 to 2000, and of Enzyme Dynamics and Regulation. Dr. Chock is a member of the American Chemical Society and the American Society for Biochemistry and Molecular Biology.
Selected Publications
- Oubrahim H, Wong A, Wilson BA, Chock PB. Mammalian Target of Rapamycin Complex 1 (mTORC1) Plays a Role in Pasteurella multocida Toxin (PMT)-induced Protein Synthesis and Proliferation in Swiss 3T3 Cells. J Biol Chem. 2013;288(4):2805-15.
- Tanaka M, Chock PB, Stadtman ER. Oxidized messenger RNA induces translation errors. Proc Natl Acad Sci U S A. 2007;104(1):66-71.
- Barrett WC, DeGnore JP, Keng YF, Zhang ZY, Yim MB, Chock PB. Roles of superoxide radical anion in signal transduction mediated by reversible regulation of protein-tyrosine phosphatase 1B. J Biol Chem. 1999;274(49):34543-6.
- Yim MB, Kang JH, Yim HS, Kwak HS, Chock PB, Stadtman ER. A gain-of-function of an amyotrophic lateral sclerosis-associated Cu,Zn-superoxide dismutase mutant: An enhancement of free radical formation due to a decrease in Km for hydrogen peroxide. Proc Natl Acad Sci U S A. 1996;93(12):5709-14.
- Wu XM, Gutfreund H, Chock PB. Kinetic method for differentiating mechanisms for ligand exchange reactions: application to test for substrate channeling in glycolysis. Biochemistry. 1992;31(7):2123-8.
Related Scientific Focus Areas
Biomedical Engineering and Biophysics
View additional Principal Investigators in Biomedical Engineering and Biophysics
Molecular Biology and Biochemistry
View additional Principal Investigators in Molecular Biology and Biochemistry
This page was last updated on Tuesday, June 10, 2025