Spell-Checking DNA
Thomas Kunkel inspects DNA replication fidelity for underpinnings of health and disease.
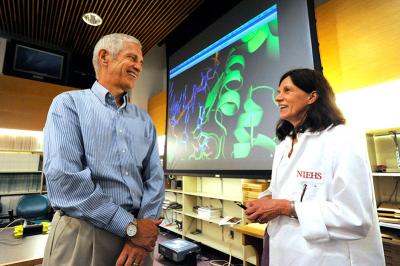
Thomas Kunkel, Ph.D., and Katarzyna Bebenek, Ph.D., discuss findings from data produced in the lab.
When discussing medical research, we often think about biological processes gone wrong—unanticipated errors that somehow need to be fixed with therapies or other interventions. Yet, the human body works almost perfectly, almost all of the time, due in part to the incredible precision achieved as cells divide and multiply.
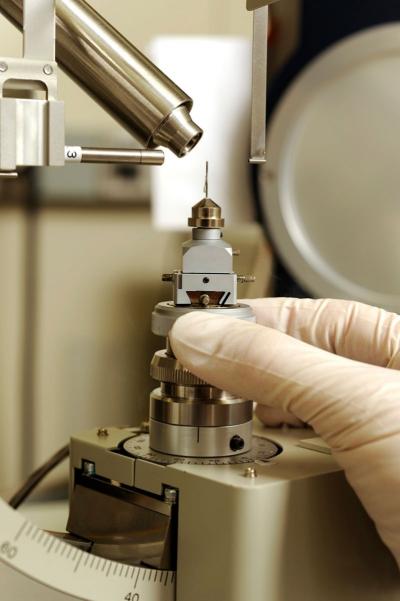
Dr. Kunkel’s team uses X-ray crystallography to determine the structures of DNA polymerases, the enzymes that replicate DNA.
For the past 40 years, Thomas Kunkel, Ph.D., has explored the intricate genetic and molecular mechanisms underlying that cornerstone of human existence. To hear Kunkel describe it, ensuring the fidelity of DNA replication employs tools not unlike those in word-processing software.
“To put it simply, the human genome contains as much information as approximately 2,000 textbooks, and a human cell can replicate—or copy—that collection of textbooks in about 8 hours,” Kunkel explains. “When we talk about fidelity of DNA replication, we’re talking about the ability to copy all 2,000 books with an estimated error rate of less than one typo. It’s incredibly accurate.”
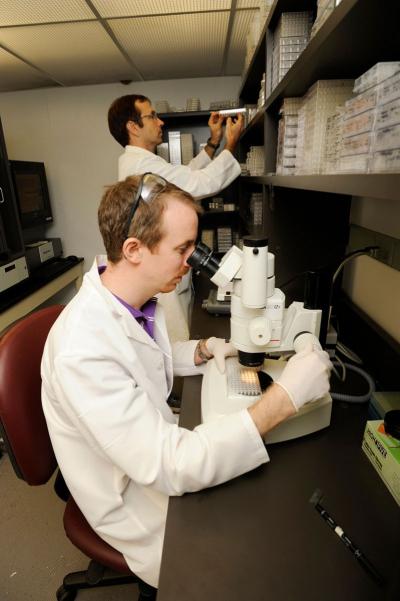
Anders Clausen, Ph.D., (front) examines crystals of DNA polymerases, while Lars Pedersen, Ph.D., stores additional samples in the process of forming crystals.
In word processing, there are three steps to ensuring accuracy. The first is the initial typing, when a person hits either the right or wrong key to type the word they wish to convey. If that person hits a wrong key, “backspace” (step two) can correct it. Finally, when the document is complete, “spell-check” (step three) confirms its accuracy.
Similar actions occur within the human genome. However, when it comes to DNA replication, a mistake in any of the steps can give rise to detrimental health effects.
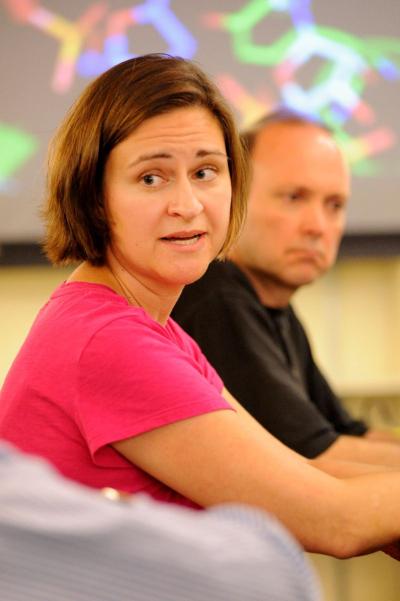
Jessica Williams, Ph.D., (front) and Alan Clark, Ph.D., participate in a lab meeting with the team.
DNA base pairing—how the building blocks of the DNA double helix bind together—is like the initial typing step. Kunkel’s team studies how and how well the pairing choice is made, what happens when the wrong choice is made, and how to correct it (comparable to the backspace key). They also study how DNA mismatch repair acts as a spell-checker to correct errors made during replication.
Failure anywhere alone the way sometimes leads to mutations and ultimately diseases such as cancer and hereditary neurodegenerative diseases. In the field of cancer, there is particular excitement about sequencing the genomes of tumor cells to identify mistakes made during replication. These studies indicates that tumors can result from defects in either backspace or spell-checking.
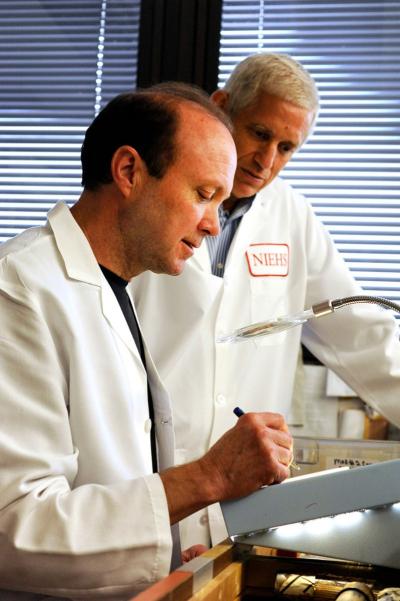
Clark (front) counts yeast cells on a petri dish, while Kunkel observes.
Kunkel and his colleagues have contributed new insights into replication fidelity in the AIDS virus, which has very low accuracy that leads to mutations that confer drug resistance. The inability of viruses to utilize backspace and spell-check functions is what makes them such tough pathogens to treat.
“But low fidelity isn’t all bad news,” Kunkel says, “and there are times when organisms, even humans, actually need an extraordinarily high mutation rate in order to maintain our health.”
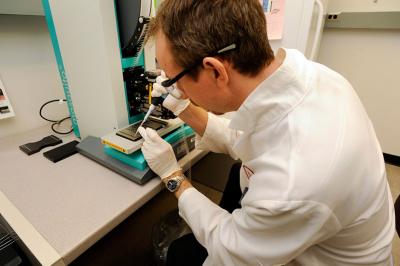
Clausen prepares protein samples for high-throughput crystallization trials to be set up by a “mosquito” robot.
When the human immune system encounters a foreign pathogen, its B cells make antibodies targeted to the pathogen, helping other cells find and destroy it. Producing uniquely specific pathogen-shaped antibodies requires a mutation rate in specific cells that is 10,000 to 1,000,000 times higher than what occurs in most cells. This controlled hypermutation is essential to the development of a normal immune system.
After four decades on the job, Kunkel still finds joy in the many surprises that basic research affords. A few summers ago, members of his lab discovered that a large number of ribonucleotides, the normal building blocks for RNA, is incorporated into the DNA genome, a result that has unanticipated implications and provides numerous opportunities for further study.
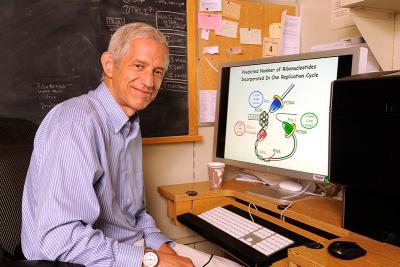
In his office, Dr. Kunkel plans new approaches to understanding the repercussions of DNA replication infidelity.
“One of the reasons that I’ve spent 31 of the last 40 years in the Intramural Research Program at the NIH is that, when we see these opportunities, we can quickly mobilize resources to investigate further,” Kunkel says.
In this case, he shifted about half of his lab to further studies of ribonucleotide incorporation during DNA replication, leading to publication of several papers describing a new aspect of genome instability. Interest in ribonucleotide incorporation into DNA continues to increase as scientists explore potential associations with neurodegenerative diseases.
Thomas Kunkel, Ph.D., is an NIH Distinguished Investigator in the Genome Integrity and Structural Biology Laboratory at the National Institute of Environmental Health Sciences (NIEHS).
*There are two deliberate typos in this article. If you can find them, let us know at IRPinfo@mail.nih.gov.
This page was last updated on Wednesday, May 24, 2023